Precise Positioning and Navigation I
Tracks
Room E3
Friday, September 6, 2019 |
9:00 AM - 10:40 AM |
Details
Chair: Prof. T. Moore (U. Nottingham)
Speaker
Attendee91
Leica Geosystems AG
Assessing the Benefits of Galileo to High-Precision GNSS – RTK, PPP and Post-Processing
Abstract Text
The declaration of Galileo Initial Services in December 2016 was the first step towards its Full Operational Capability (FOC) by 2020. On February 11, 2019 the latest batch of four Galileo satellites has been commissioned for operational use, accelerating the evolution of the Galileo constellation towards FOC. With 22 satellites now in service, all GNSS users worldwide will not only be able to benefit from Galileo signals in combination with other constellations, but the possibility of using Galileo as a stand-alone system also increases. In the case of high-precision GNSS including RTK (real-time kinematic), PPP (precise point positioning) and post-processing positioning, improved availability, accuracy and reliability are highly expected from the user perspective through Galileo integration.
To fully assess the benefits of Galileo signals, GNSS receivers and post-processing software products require sophisticated algorithms, which provide not only robust multi-constellation solutions, but also reliable positions from the individual satellite systems. All the Galileo signals in the E1 and E5 frequency band have been successfully integrated in Leica Geosystems’ real-time (RTK and PPP) and post-processing GNSS technologies. In terms of RTK, multi-frequency linear combination and advanced decorrelation techniques are extended for Galileo. With regard to PPP, the Leica SmartLink service is used, which supports the GPS, GLONASS, Galileo and BeiDou constellations. The ambiguity estimation in PPP is performed by means of a more sophisticated method referred to as the best integer equivariant (BIE) approach. For post-processing applications Galileo is also involved, where the reference station data can be either observed or virtual, through the creation of virtual RINEX by exploiting the HxGN SmartNet service.
This paper especially addresses the benefits of Galileo to high-precision GNSS positioning in real-time and post-processing mode. Based on representative data sets collected under different observational conditions, the contribution of the current Galileo constellation to overall satellite geometry is assessed. With a special focus on challenging environments like urban canyons and dense canopy, the benefits of Galileo to RTK are analysed with respect to availability, accuracy and reliability. The results show that the incorporation of Galileo satellites and signals improves the RTK fix availability, providing more positions with centimetre-level accuracy. Moreover, the occurrence of false ambiguity fix can be effectively reduced. In addition to multi-GNSS solution, the performance of Galileo-only RTK is also evaluated. The PPP-related analyses demonstrate how Galileo benefits the convergence time and positioning quality. By applying the BIE ambiguity estimator together with the SmartLink service, the initial convergence time to full PPP accuracy (i.e. 3 cm in 2D) is significantly reduced by up to 40%. The post-processing studies are carried out with different baseline lengths and data acquisition conditions. The advantages of Galileo integration are investigated in the observation and position domains, using virtual and physical reference station.
To fully assess the benefits of Galileo signals, GNSS receivers and post-processing software products require sophisticated algorithms, which provide not only robust multi-constellation solutions, but also reliable positions from the individual satellite systems. All the Galileo signals in the E1 and E5 frequency band have been successfully integrated in Leica Geosystems’ real-time (RTK and PPP) and post-processing GNSS technologies. In terms of RTK, multi-frequency linear combination and advanced decorrelation techniques are extended for Galileo. With regard to PPP, the Leica SmartLink service is used, which supports the GPS, GLONASS, Galileo and BeiDou constellations. The ambiguity estimation in PPP is performed by means of a more sophisticated method referred to as the best integer equivariant (BIE) approach. For post-processing applications Galileo is also involved, where the reference station data can be either observed or virtual, through the creation of virtual RINEX by exploiting the HxGN SmartNet service.
This paper especially addresses the benefits of Galileo to high-precision GNSS positioning in real-time and post-processing mode. Based on representative data sets collected under different observational conditions, the contribution of the current Galileo constellation to overall satellite geometry is assessed. With a special focus on challenging environments like urban canyons and dense canopy, the benefits of Galileo to RTK are analysed with respect to availability, accuracy and reliability. The results show that the incorporation of Galileo satellites and signals improves the RTK fix availability, providing more positions with centimetre-level accuracy. Moreover, the occurrence of false ambiguity fix can be effectively reduced. In addition to multi-GNSS solution, the performance of Galileo-only RTK is also evaluated. The PPP-related analyses demonstrate how Galileo benefits the convergence time and positioning quality. By applying the BIE ambiguity estimator together with the SmartLink service, the initial convergence time to full PPP accuracy (i.e. 3 cm in 2D) is significantly reduced by up to 40%. The post-processing studies are carried out with different baseline lengths and data acquisition conditions. The advantages of Galileo integration are investigated in the observation and position domains, using virtual and physical reference station.
Attendee83
Swiss Federal Office of Topography swisstopo
Impact of Multi-GNSS observations on precise geodetic applications used at the Swiss Mapping Agency swisstopo
Abstract Text
Today, many GNSS permanent networks are enhanced from GPS or GPS/GLONASS networks to multi-GNSS networks supporting also the signals of the European Galileo and the Chinese BeiDou satellite systems. The additional signals are beneficial to many applications. This paper focuses on the impact on precise geodetic applications, such as precise coordinate determination based on daily solutions and also on improvements of precise kinematic PPP solutions. Here, results from the complete processing chain are shown – from data collection to analysis.
swisstopo, the Swiss Mapping agency, enhanced its permanent network to multi-GNSS beginning 2015. A complete multi-GNSS data flow based on RINEX3 was established parallel to the RINEX2 data flow in mid-2015. In parallel to these developments, also the field equipment was replaced after conducting a technical evaluation. All 200 reference points of the Swiss terrestrial reference frame were re-measured in 2016 by static multi-GNSS observations of about 48 hours per station.
On the analysis side, the scientific Bernese GNSS Software was updated enabling multi-GNSS capability and making use of the IGS MGEX products (especially the CODE-derived multi-GNSS orbits). These developments were used to analyse the multi-GNSS data from the field campaigns as well as to analyse the permanent networks. As first analysis centre in Europe, swisstopo switched the contribution of daily and weekly solutions to the European Permanent Network (EPN) of EUREF (IAG sub-commission on Reference Frames in Europe) to multi-GNSS in mid-2016. We headline also the progress achieved concerning multi-GNSS within EUREF.
Intersystem parameters are an important indicator of the compatibility of the coordinates and troposphere parameters between the various satellite systems. Examples will show the order of magnitude these parameters can reach and will demonstrate its impact on the final results.
On the way towards real-time, we show results of kinematic PPP solutions, addressing the possible gain using additional satellite systems. Finally, we show the status of using multi-GNSS data in the real-time positioning service swipos.
swisstopo, the Swiss Mapping agency, enhanced its permanent network to multi-GNSS beginning 2015. A complete multi-GNSS data flow based on RINEX3 was established parallel to the RINEX2 data flow in mid-2015. In parallel to these developments, also the field equipment was replaced after conducting a technical evaluation. All 200 reference points of the Swiss terrestrial reference frame were re-measured in 2016 by static multi-GNSS observations of about 48 hours per station.
On the analysis side, the scientific Bernese GNSS Software was updated enabling multi-GNSS capability and making use of the IGS MGEX products (especially the CODE-derived multi-GNSS orbits). These developments were used to analyse the multi-GNSS data from the field campaigns as well as to analyse the permanent networks. As first analysis centre in Europe, swisstopo switched the contribution of daily and weekly solutions to the European Permanent Network (EPN) of EUREF (IAG sub-commission on Reference Frames in Europe) to multi-GNSS in mid-2016. We headline also the progress achieved concerning multi-GNSS within EUREF.
Intersystem parameters are an important indicator of the compatibility of the coordinates and troposphere parameters between the various satellite systems. Examples will show the order of magnitude these parameters can reach and will demonstrate its impact on the final results.
On the way towards real-time, we show results of kinematic PPP solutions, addressing the possible gain using additional satellite systems. Finally, we show the status of using multi-GNSS data in the real-time positioning service swipos.
Attendee81
Leibniz University Hannover
Improved Carrier Phase-based GNSS Position and Velocity Determination Using a Transportable Passive Hydrogen Maser
Abstract Text
Standalone navigation using GNSS (Global Navigation Satellite System) measurements always requires the synchronization of satellite and receiver timescales, which is usually realized by introducing so-called clock errors relative to GNSS time. In high-precision applications such as precise point positioning (PPP), satellite clock corrections are derived from clock products of the International GNSS Service. On the receiver side, the clock error must be corrected by the user, typically by estimating an additional parameter together with the user coordinates. The same applies to velocity determination of the user. Due to the poor accuracy and limited long-term stability of the internal quartz oscillator of the receiver, this error source has to be estimated on an epoch-wise basis. This impacts the whole observation geometry in three ways: (a) at least four satellites must be observed simultaneously, (b) the up-component is estimated less precisely than the horizontal components, and (c) significant correlations between the receiver clock error, the up-coordinate and other elevation-dependent errors such as the tropospheric delay arise.
Replacing the internal oscillator by a much more stable external atomic clock and introducing the knowledge about the superior accuracy and stability of such an oscillator into the estimation process, opens up the possibility of applying receiver clock modeling (RCM). This approach is physically meaningful over time intervals during which the clock noise is well below the receiver noise. Since the latter is dominated by the observation type in use, RCM is restricted to code observations when using a chip-scale atomic clock (CSAC) as external oscillator. Thus, the use of carrier phase observations, like e.g., in PPP, is only possible when an oscillator of much higher stability is connected to the receiver. The theoretically required frequency stability for this purpose can be provided by a transportable passive hydrogen maser (PHM), for example the Vremya VCH-1006.
In previous contributions, we already discussed the impact of such a high-precision oscillator on kinematic PPP. For this purpose an automotive experiment was carried out in the city of Hannover, Germany, using a PHM onboard a van, where the maser was connected to a geodetic receiver. The experiment lasted for approximately 75 minutes and was about 20 km long covering both urban areas and city highways. Preliminary results showed improvements of the precision of the vertical coordinate and velocity estimates of roughly 60-70 percent when applying RCM. This also lead to better reliability and integrity of the PPP solution. Although no significant impact on the convergence time of the real-valued ambiguities could be found, further investigations in this regard were deemed necessary, and their results will be presented here. In addition, we will discuss the Kalman filter-based analysis of the complete automotive experiment. Here, the main focus will be on the impact of PPP clock modeling in different environmentally challenging scenarios such as urban canyons and open sky areas. Since such an oscillator is not primarily designed to be used in such an experiment, we will also discuss the impact of external influences such as vibrations on the PPP solution.
Replacing the internal oscillator by a much more stable external atomic clock and introducing the knowledge about the superior accuracy and stability of such an oscillator into the estimation process, opens up the possibility of applying receiver clock modeling (RCM). This approach is physically meaningful over time intervals during which the clock noise is well below the receiver noise. Since the latter is dominated by the observation type in use, RCM is restricted to code observations when using a chip-scale atomic clock (CSAC) as external oscillator. Thus, the use of carrier phase observations, like e.g., in PPP, is only possible when an oscillator of much higher stability is connected to the receiver. The theoretically required frequency stability for this purpose can be provided by a transportable passive hydrogen maser (PHM), for example the Vremya VCH-1006.
In previous contributions, we already discussed the impact of such a high-precision oscillator on kinematic PPP. For this purpose an automotive experiment was carried out in the city of Hannover, Germany, using a PHM onboard a van, where the maser was connected to a geodetic receiver. The experiment lasted for approximately 75 minutes and was about 20 km long covering both urban areas and city highways. Preliminary results showed improvements of the precision of the vertical coordinate and velocity estimates of roughly 60-70 percent when applying RCM. This also lead to better reliability and integrity of the PPP solution. Although no significant impact on the convergence time of the real-valued ambiguities could be found, further investigations in this regard were deemed necessary, and their results will be presented here. In addition, we will discuss the Kalman filter-based analysis of the complete automotive experiment. Here, the main focus will be on the impact of PPP clock modeling in different environmentally challenging scenarios such as urban canyons and open sky areas. Since such an oscillator is not primarily designed to be used in such an experiment, we will also discuss the impact of external influences such as vibrations on the PPP solution.
Attendee155
Deimos Space
HD-GNSS: real-time absolute navigation at sub-centimetre level
Abstract Text
Currently GNSS Systems provide instantaneous absolute positioning accuracy of a few meters (assuming no local obstructions above 5-10 degrees elevation) based on the navigation signals broadcast by a MEO constellation. This positioning accuracy can be improved extraordinarily by means of the GNSS evolutions, such as the augmentation of the Space Segment and Ground Segment, the enhancement of the payload output signals stability, the introduction of new technologies such as Inter-Satellite Link (ISL), the definition and implementation of algorithms for generating and processing additional and more accurate information, such as multi-carrier ambiguity resolution (MCAR) techniques.
In this evolving background, studies to demonstrate System concept feasibility are on-going. In particular the HDGNSS (High Definition GNSS), led by Deimos Space (DMS) in cooperation with Delft University of Technology (TUD) and Technische Universität München (TUM) in the frame of ESA’s General Studies Programme (GSP), investigates the feasibility of having real-time GNSS-based absolute navigation at sub-centimetre level (error < 1 cm in the position domain) by new generation of GNSS System and new generation of GNSS User Equipment.
The project, now entering the experimentation phase, studies the development of a PPP-RTK-based high precision service for European users by applying state-of-the-art algorithms for both the network and the user platforms and by investigating multi-frequency and multi-GNSS solutions.
The PPP-RTK concept consists in providing users with precise GNSS correction data so as to enable integer ambiguity resolution and consequently fast convergence. In the HDGNSS study, a PPP-RTK model based on the processing of undifferenced and uncombined code and phase observables is applied which makes use of Common Clock S-basis.
An end-to-end approach is adopted to define requirements for both network and user platforms.
On the user side, the required corrections quality is determined for compliance with the performance targets of positioning precision, time-to-first-fix, and success rate for ambiguity fixing, aiming at real-time sub-centimetre level positioning. An in-house developed MCAR engine is used to demonstrate single constellation performance at user level, and the performance improvement from using regional augmentation satellites and/or additional constellations are assessed.
On the system side, the network architecture is defined that enables the estimation and dissemination of such corrections. Two overlapping networks are considered for PPP products estimation, a global network for estimation of the satellite parameters and a regional (over Europe) network for the generation of ionospheric delays corrections. Availability of precise information about the satellite orbits and stations positions is assumed. A covariance analysis approach is used to dimension the two networks in different scenarios, namely for different station types and for varying orbit products accuracy.
The experimentation campaign will evaluate the end-to-end HD-GNSS performance, providing significant information for consolidating the final system design in different experimentation scenarios. Most significant results will be presented in the current paper.
In this evolving background, studies to demonstrate System concept feasibility are on-going. In particular the HDGNSS (High Definition GNSS), led by Deimos Space (DMS) in cooperation with Delft University of Technology (TUD) and Technische Universität München (TUM) in the frame of ESA’s General Studies Programme (GSP), investigates the feasibility of having real-time GNSS-based absolute navigation at sub-centimetre level (error < 1 cm in the position domain) by new generation of GNSS System and new generation of GNSS User Equipment.
The project, now entering the experimentation phase, studies the development of a PPP-RTK-based high precision service for European users by applying state-of-the-art algorithms for both the network and the user platforms and by investigating multi-frequency and multi-GNSS solutions.
The PPP-RTK concept consists in providing users with precise GNSS correction data so as to enable integer ambiguity resolution and consequently fast convergence. In the HDGNSS study, a PPP-RTK model based on the processing of undifferenced and uncombined code and phase observables is applied which makes use of Common Clock S-basis.
An end-to-end approach is adopted to define requirements for both network and user platforms.
On the user side, the required corrections quality is determined for compliance with the performance targets of positioning precision, time-to-first-fix, and success rate for ambiguity fixing, aiming at real-time sub-centimetre level positioning. An in-house developed MCAR engine is used to demonstrate single constellation performance at user level, and the performance improvement from using regional augmentation satellites and/or additional constellations are assessed.
On the system side, the network architecture is defined that enables the estimation and dissemination of such corrections. Two overlapping networks are considered for PPP products estimation, a global network for estimation of the satellite parameters and a regional (over Europe) network for the generation of ionospheric delays corrections. Availability of precise information about the satellite orbits and stations positions is assumed. A covariance analysis approach is used to dimension the two networks in different scenarios, namely for different station types and for varying orbit products accuracy.
The experimentation campaign will evaluate the end-to-end HD-GNSS performance, providing significant information for consolidating the final system design in different experimentation scenarios. Most significant results will be presented in the current paper.
Attendee203
Federal Office Of Topography Swisstopo
Robot field calibration for multi-GNSS receiver antennas at ETH Zurich
Abstract Text
Introduction
GNSS antennas suffer from direction-dependent errors, which are due to the physical properties of the antenna. These errors affect the satellite transmitter antennas as well as the receiver antennas. The transmitter antennas of the Galileo spacecraft were precisely calibrated in specifically designed anechoic chambers before the launch of the spacecraft. The calibrations are publicly available. In order to exploit the full potential of the Galileo constellation, receiver antennas should be calibrated as well.
State of the art
Antenna calibrations have been studied since the very beginning of GPS in the 80s, for instance by Sims (1985). Nowadays, two methods are well established: the calibration in anechoic chambers using an artificial signal source (Zeimetz 2002) and the robot calibration in the field using real GNSS signals (Willi 2019, Menge 2003).
In the case of the robot calibration, a device is used to rotate the antenna in the field while real GNSS data is acquired. This is necessary, in order to de-correlate the Phase Center Corrections (PCC) from the Phase Center Offsets (PCO) and from multipath effects. Typically, an industrial robot with five or six axes is used to rotate the receiver antenna.
Various groups perform field calibrations with industrial robots. The most prominent system was developed at the Leibniz University Hannover (Menge 2003) and is now commercially operated by the German company Geo++ (Wübbena et al. 2000). Currently, this system is able to deliver calibration values for the GPS L1 and L2 and the GLONASS G1 and G2 frequencies. An implementation of Galileo E5 calibrations has been announced for 2019 . Other groups are working on antenna field calibrations as well (Bilich et al. 2018, Hu et al. 2015, Riddell et al. 2015), but none of them reportedly performed calibrations for Galileo signals.
Receiver antenna calibration at ETH Zurich
The Institute of Geodesy and Photogrammetry at ETH Zurich developed a field calibration method for GNSS receiver antennas (Willi 2019). The system uses a six axes industrial robot of brand KUKA. One calibration sequence consists of 2000 to 4000 antenna orientations and lasts for 1-4 hours. The acquired data is then processed in a triple-difference approach (Willi et al. 2018). In principle, the system is able to generate PCC for all Code Division Multiple Access (CDMA) signals. It has been tested so far for GPS and Galileo.
Anticipated results
In order to validate the approach, a validation dataset has been acquired on short baselines. Four antennas were placed on four geodetic pillars for at least 24 h. In total, four sessions were measured, permuting the antennas between the different sessions. The coordinates of the geodetic pillars were precisely surveyed beforehand, resulting in a very accurate ground truth (standard deviation of about 0.2 mm). The GNSS data was then processed with a development version of the Bernese GNSS Software (Dach et al. 2015). In this contribution, the results from this campaign will be presented and discussed in detail, as well as the resulting consequences for the Galileo science community.
GNSS antennas suffer from direction-dependent errors, which are due to the physical properties of the antenna. These errors affect the satellite transmitter antennas as well as the receiver antennas. The transmitter antennas of the Galileo spacecraft were precisely calibrated in specifically designed anechoic chambers before the launch of the spacecraft. The calibrations are publicly available. In order to exploit the full potential of the Galileo constellation, receiver antennas should be calibrated as well.
State of the art
Antenna calibrations have been studied since the very beginning of GPS in the 80s, for instance by Sims (1985). Nowadays, two methods are well established: the calibration in anechoic chambers using an artificial signal source (Zeimetz 2002) and the robot calibration in the field using real GNSS signals (Willi 2019, Menge 2003).
In the case of the robot calibration, a device is used to rotate the antenna in the field while real GNSS data is acquired. This is necessary, in order to de-correlate the Phase Center Corrections (PCC) from the Phase Center Offsets (PCO) and from multipath effects. Typically, an industrial robot with five or six axes is used to rotate the receiver antenna.
Various groups perform field calibrations with industrial robots. The most prominent system was developed at the Leibniz University Hannover (Menge 2003) and is now commercially operated by the German company Geo++ (Wübbena et al. 2000). Currently, this system is able to deliver calibration values for the GPS L1 and L2 and the GLONASS G1 and G2 frequencies. An implementation of Galileo E5 calibrations has been announced for 2019 . Other groups are working on antenna field calibrations as well (Bilich et al. 2018, Hu et al. 2015, Riddell et al. 2015), but none of them reportedly performed calibrations for Galileo signals.
Receiver antenna calibration at ETH Zurich
The Institute of Geodesy and Photogrammetry at ETH Zurich developed a field calibration method for GNSS receiver antennas (Willi 2019). The system uses a six axes industrial robot of brand KUKA. One calibration sequence consists of 2000 to 4000 antenna orientations and lasts for 1-4 hours. The acquired data is then processed in a triple-difference approach (Willi et al. 2018). In principle, the system is able to generate PCC for all Code Division Multiple Access (CDMA) signals. It has been tested so far for GPS and Galileo.
Anticipated results
In order to validate the approach, a validation dataset has been acquired on short baselines. Four antennas were placed on four geodetic pillars for at least 24 h. In total, four sessions were measured, permuting the antennas between the different sessions. The coordinates of the geodetic pillars were precisely surveyed beforehand, resulting in a very accurate ground truth (standard deviation of about 0.2 mm). The GNSS data was then processed with a development version of the Bernese GNSS Software (Dach et al. 2015). In this contribution, the results from this campaign will be presented and discussed in detail, as well as the resulting consequences for the Galileo science community.
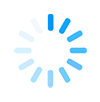