Fundamental Physics II
Tracks
Room E3
Thursday, September 5, 2019 |
11:10 AM - 12:50 PM |
Details
Chair: Dr. F. Vespe (Space Geodetic Centre, ASI)
Speaker
Attendee167
Observatoire De Paris, Sorbonne Université
A gravitational redshift test using eccentric Galileo satellites
Abstract Text
We present the results of the analysis of the GREAT (Galileo gravitational Redshift test with Eccentric sATellites) experiment from SYRTE (Observatoire de Paris), funded by the European Space Agency. An elliptic orbit induces a periodic modulation of the fractional frequency difference between a ground clock and the satellite clock, while the good stability of Galileo clocks allows to test this periodic modulation to a high level of accuracy. Galileo 201 and 202, with their large eccentricity and on-board H-maser clocks, are perfect candidates to perform this test. SLR data allows us to partly decorrelate the orbit perturbations from the clock errors. By analyzing several years of Galileo satellites data we have been able to improve on the GP-A test of the gravitational redshift.
Attendee140
Universität Bremen
Testing General Relativity with GSAT-0201 and GSAT-0202
Abstract Text
We present the results from an analysis of three years of clock and orbit data from GSAT-0201 and 0202, which have erroneously been injected into an eccentric orbit in 2014. Aiming to do a sensitive test of the gravitational redshift of the onboard clocks as predicted by general relativity, the clock and orbit data from these satellites has been preprocessed and made available to us through the ESA/ESOC Navigation Support Office in the framework of the ESA-led GREAT project. The results of our analysis have been published end of December 2018 in Physical Review Letters, claiming a more than four-fold improvement over the most accurate redshift test so far, as obtained by the Gravity Probe A mission.
In this talk, we will describe the approach chosen for the data analysis, discuss the systematic uncertainties we have identified and the limitations they impose on our result. In total, we include the data of three Passive Hydrogen Masers into our analysis, with the best results obtained from PHM-A on GSAT-0202. Further, we have identified mismodelling of solar radiation pressure to be one of the leading contributions to the systematic error. This effect could be significantly reduced, thanks to the use of satellite laser ranging data as a mean to independently assess the magnitude of orbit model errors.
Finally, we will summarize the lessons learned from our analysis and discuss future perspectives on consolidating and improving these results. PHM-A on GSAT-0202 is continuously producing further clock data that could be used for such further analysis, possibly aided by telemetry data on temperature and clock operations. Also we are working on an improved a priori SRP model, based on finite element analysis of a geometrical model of the satellites. Evaluation of this SRP model in orbit modeling shows promising results and we think that this model may be useful also in further applications.
In this talk, we will describe the approach chosen for the data analysis, discuss the systematic uncertainties we have identified and the limitations they impose on our result. In total, we include the data of three Passive Hydrogen Masers into our analysis, with the best results obtained from PHM-A on GSAT-0202. Further, we have identified mismodelling of solar radiation pressure to be one of the leading contributions to the systematic error. This effect could be significantly reduced, thanks to the use of satellite laser ranging data as a mean to independently assess the magnitude of orbit model errors.
Finally, we will summarize the lessons learned from our analysis and discuss future perspectives on consolidating and improving these results. PHM-A on GSAT-0202 is continuously producing further clock data that could be used for such further analysis, possibly aided by telemetry data on temperature and clock operations. Also we are working on an improved a priori SRP model, based on finite element analysis of a geometrical model of the satellites. Evaluation of this SRP model in orbit modeling shows promising results and we think that this model may be useful also in further applications.
Attendee167
Observatoire De Paris, Sorbonne Université
Chronometric geodesy: geopotential determination using clock comparisons
Abstract Text
At the beginning of the 20th century the theories of special and general relativity were developed by Einstein and his contemporaries. These physical theories revolutionize our conceptions of time and of the measurement of time. The atomic clocks, which appeared in the 1950s, are so accurate and stable that it is now essential to take into account many relativistic effects. The development and worldwide comparisons of such atomic clocks allowed for some of the most stringent of fundamental physics, as well as new ideas for the search of dark matter. On a more applied level, when taking general relativity for granted, distant comparisons of atomic clocks can be used for navigation and positioning, as well as the determination of the geopotential. I will show how the chronometric observables can fit and be used within the context of classical geodesy.
Attendee72
Universitat Politècnica de Catalunya (UPC)
New approach for computing satellite clocks focused on testing general relativity with Galileo satellites
Abstract Text
The estimation of the fractional part of carrier phase ambiguities (also named phase biases) for satellites and receivers is a key point in order to fix carrier phase ambiguities when processing un-differenced measurements. Initially, phase biases were introduced in order to allow users to achieve similar accuracies using PPP than those using differential techniques, as RTK, with the advantage that PPP can be applied globally, while RTK can only be applied over a regional area.
In the context of the ongoing ESA project General Relativity Experiment (GREAT), we are developing a new strategy to estimate accurate satellite clocks, which are the key element for testing the general relativity predictions, as well as other fundamental aspects of sciences.
In this work we show how phase bias estimates, i.e. unambiguous carrier phases, can also help to compute satellite corrections (in general) and clocks in particular. Indeed, based on the phase biases estimates, we present a new approach for computing satellite and receiver clocks that involves all the frequencies, instead of using just the ionospheric free combination, which is the usual way for estimating satellite and receiver clocks. Therefore, we are able to estimate, in the same process, satellite and receiver clocks and the different inter-frequency and inter-constellation biases.
We apply this new strategy for estimating clock and biases for receivers and for GPS and Galileo satellites, taking benefit from the stability of H-maser frequency standard on board of the Galileo satellites. The period considered in study involves 16 days of 2017, from day of year 284 to 299. During these days, the Galileo constellation had 17 satellites, which was enough to apply the strategy.
The main results can be summarized as:
Unambiguous carrier phase measurements (i.e. very precise pseudoranges) can be obtained during the whole period of 16 days. This is thanks to the stability of the carrier phase biases during the whole period. As a consequence, and in spite each day the data is processed independently, the unambiguous phase measurements do not present discontinuities between days.
While in the standard processing of combined GPS and Galileo data, clock daily solutions presents small jumps from day to day estimates (at the level of 0.1 ns for GPS and several tenths of a nanoseconds for Galileo), with our strategy, these jumps practically disappear thanks to the continuity of the phase measurements.
The phase biases estimates, which in our strategy are done together with clocks, exhibit temperature depend variation for the L5 frequency of the GPS block IIF. This dependency was reported by some authors using geometry free combinations.
Finally, taking advantage from the high stability of the clocks on board of Galileo satellites, clock solutions using several strategies will be compared. From these comparisons, we will discuss how general relativity tests can benefit from these improved clock estimates.
In the context of the ongoing ESA project General Relativity Experiment (GREAT), we are developing a new strategy to estimate accurate satellite clocks, which are the key element for testing the general relativity predictions, as well as other fundamental aspects of sciences.
In this work we show how phase bias estimates, i.e. unambiguous carrier phases, can also help to compute satellite corrections (in general) and clocks in particular. Indeed, based on the phase biases estimates, we present a new approach for computing satellite and receiver clocks that involves all the frequencies, instead of using just the ionospheric free combination, which is the usual way for estimating satellite and receiver clocks. Therefore, we are able to estimate, in the same process, satellite and receiver clocks and the different inter-frequency and inter-constellation biases.
We apply this new strategy for estimating clock and biases for receivers and for GPS and Galileo satellites, taking benefit from the stability of H-maser frequency standard on board of the Galileo satellites. The period considered in study involves 16 days of 2017, from day of year 284 to 299. During these days, the Galileo constellation had 17 satellites, which was enough to apply the strategy.
The main results can be summarized as:
Unambiguous carrier phase measurements (i.e. very precise pseudoranges) can be obtained during the whole period of 16 days. This is thanks to the stability of the carrier phase biases during the whole period. As a consequence, and in spite each day the data is processed independently, the unambiguous phase measurements do not present discontinuities between days.
While in the standard processing of combined GPS and Galileo data, clock daily solutions presents small jumps from day to day estimates (at the level of 0.1 ns for GPS and several tenths of a nanoseconds for Galileo), with our strategy, these jumps practically disappear thanks to the continuity of the phase measurements.
The phase biases estimates, which in our strategy are done together with clocks, exhibit temperature depend variation for the L5 frequency of the GPS block IIF. This dependency was reported by some authors using geometry free combinations.
Finally, taking advantage from the high stability of the clocks on board of Galileo satellites, clock solutions using several strategies will be compared. From these comparisons, we will discuss how general relativity tests can benefit from these improved clock estimates.
Attendee38
Wroclaw University of Environmental and Life Sciences
Measurements of the Galileo orbit geometry deformations caused by the general relativity
Abstract Text
Two Galileo satellites launched into highly-eccentric orbits constitute perfect probes for the verification of effects emerging from the general relativity. The observation of the general relativity effects affecting the Galileo clocks demonstrated an exceptional accuracy when compared to previous missions dedicated to the verification of the relativistic effects, such as Gravity Probe-B. The impact of the general relativity effects including the Schwarzschild, Lense-Thirring, and DeSitter effects on the deformation of satellite geometry has not, however, been studied so far. The size of the orbit can be characterized by the semi-major axis, whereas the shape of the orbit can be characterized by the orbital eccentricity. We show how the size and the shape of Galileo orbits deform due to three effects emerging from general relativity on the example of Galileo satellites in nominal orbits and two Galileo E14 and E18 launched into high-eccentric orbits whose differences between the perigee and apogee heights are 8800 km. We derive precise Galileo orbits based on the box-wing model for solar radiation pressure, Earth’s albedo, infrared radiation, antenna thrust and a simple model for the misalignment of solar panels in two dimensions with no empirical periodic accelerations additionally estimated. The box-wing model guarantees a very high quality of determined orbits and allows for analyzing small-scale perturbing effects. One solution is generated using the classical Newtonian formalism and the second employs the parameterized post-Newtonian formalism for the Schwarzschild, Lense-Thirring (frame dragging), and DeSitter (geodetic precession) effects using a global network of multi-GNSS stations tracking Galileo satellites.
We show that the change of the satellite osculating semi-major axis due to the Schwarzschild effect depends on the satellite position and equals 27 mm when E14/E18 are in their perigees and 10 mm when satellites are in the apogees. However, the change of the satellite height, i.e., the radial component, due to the Schwarzschild effect is, paradoxically, the same for all Galileo satellites, and for all heights independently whether E14 and E18 satellites are in their perigees or apogees. The constant shift of the height together with the periodic change of the semi-major axis can be explained by the fact that the orbital eccentricity, i.e., the shape of the orbit, is continuously deformed by the general relativity with a maximum deformation in the perigee. The constant change of the radial component is four times smaller than the mean change of the osculating semi-major axis and equals 3.8 mm for all Galileo satellites. The geodetic precession causes not only a secular drift of the ascending node but also a constant change of the satellite eccentricity which is independent of the satellite height. The geodetic precession introduces also a periodic change of the satellite height with a difference of 6 mm between the apogee and perigee, whereas a change of the semi-major axis is currently undetectable.
We show that the change of the satellite osculating semi-major axis due to the Schwarzschild effect depends on the satellite position and equals 27 mm when E14/E18 are in their perigees and 10 mm when satellites are in the apogees. However, the change of the satellite height, i.e., the radial component, due to the Schwarzschild effect is, paradoxically, the same for all Galileo satellites, and for all heights independently whether E14 and E18 satellites are in their perigees or apogees. The constant shift of the height together with the periodic change of the semi-major axis can be explained by the fact that the orbital eccentricity, i.e., the shape of the orbit, is continuously deformed by the general relativity with a maximum deformation in the perigee. The constant change of the radial component is four times smaller than the mean change of the osculating semi-major axis and equals 3.8 mm for all Galileo satellites. The geodetic precession causes not only a secular drift of the ascending node but also a constant change of the satellite eccentricity which is independent of the satellite height. The geodetic precession introduces also a periodic change of the satellite height with a difference of 6 mm between the apogee and perigee, whereas a change of the semi-major axis is currently undetectable.
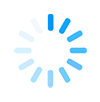