Geodesy
Tracks
Room E3
Thursday, September 5, 2019 |
3:50 PM - 5:30 PM |
Details
Chair: Dr. R. Zandbergen (ESA/ESOC)
Speaker
Attendee128
Space Research Institute, Austrian Academy of Sciences
Galileo attitude determination via high resolution satellite laser ranging
Abstract Text
Satellite laser ranging (SLR) measures the distance to satellites carrying retroreflectors with single-shot accuracies of 2-3 millimeters. SLR stations fire picosecond laser pulses to satellites; retroreflectors send the light back to the direction of the station. The reflected light is gathered by a receiving telescope and detected by a single-photon sensitive detector. Measuring the start and stop pulse of the leaving and arriving laser photons a precise time-of-fight measurement allows to calculate the range between station and satellite. When using satellite laser raging in the single-photon regime photons statistically come from all retroreflectors of the satellite. Hence kHz data delivers additional information on the reflector geometry.
We demonstrate a unique method for attitude determinatation to Galileo satellites by using high-resolution satellite laser ranging data. Ground-based distance measurements were performed to a spare panel which was mouted on a tripod 32 km outside the SLR station Graz. The panel was tilted by an angle of a maximum of 18°. For incident angles larger than 8° fine structures within the full rate data appeared. This structures were found to be directly connected to the individual columns of the retroreflectors within the panel. Determining the distance between these columns allows to directly verify the incident angle of the laser beam on the Galileo panel.
The same results were successfully achieved for a pass of Galileo 103. A specific pass was selected from orbital predictions matching the incident angle criteria as defined within the ground-based experiment. As seen from the Galileo panel reference frame (including yaw steering) Graz SLR station appears at azimuth angles of approx. -90° and elevations of 78.63°. This corresponds to a laser beam incident angle of 11.37°. SLR measurements were evaluated for an interval of approximately 400 seconds. A histogram analysis clearly showed the 11 different columns of retroreflectors. By averaging the distance between the columns the laser beam incidence angle was calculated to be 11.38°, which perfectly matches the predicted value.
We demonstrate a unique method for attitude determinatation to Galileo satellites by using high-resolution satellite laser ranging data. Ground-based distance measurements were performed to a spare panel which was mouted on a tripod 32 km outside the SLR station Graz. The panel was tilted by an angle of a maximum of 18°. For incident angles larger than 8° fine structures within the full rate data appeared. This structures were found to be directly connected to the individual columns of the retroreflectors within the panel. Determining the distance between these columns allows to directly verify the incident angle of the laser beam on the Galileo panel.
The same results were successfully achieved for a pass of Galileo 103. A specific pass was selected from orbital predictions matching the incident angle criteria as defined within the ground-based experiment. As seen from the Galileo panel reference frame (including yaw steering) Graz SLR station appears at azimuth angles of approx. -90° and elevations of 78.63°. This corresponds to a laser beam incident angle of 11.37°. SLR measurements were evaluated for an interval of approximately 400 seconds. A histogram analysis clearly showed the 11 different columns of retroreflectors. By averaging the distance between the columns the laser beam incidence angle was calculated to be 11.38°, which perfectly matches the predicted value.
Attendee125
ETH Zurich
Assessment of Differencing Strategies for SLR to GNSS satellites
Abstract Text
A well-known strategy in GNSS processing, the use of single- and double-differences, has proven to be a suitable alternative to mitigate and eliminate error sources, as well as an adequate approach to reduce the number of parameters (especially clock corrections) during the estimation process. In contrast, the traditional SLR observation processing is exclusively based on so-called zero-difference processing, where only measurements from a single receiver to a single satellite are involved. The advantage of forming single- or double-differences is the elimination (or strong reduction) of satellite- (single differences) and station-specific (double-differences) error sources and the high-accuracy achieved for short baselines. Therefore, applying these methods to SLR observations results in an excellent tool to assess existing satellite- and station-specific biases in SLR, and to estimate accurate relative coordinates between neighbouring telescopes, an important approach to validate local ties.
The very short baseline formed by the two co-located SLR telescopes (WLRS and SOS-W) at the Geodetic Observatory in Wettzell (Germany) and the quasi-simultaneous tracking of several GNSS satellites provides the opportunity to test these new observation and processing strategies in SLR, enabling the analysis of instrumental biases and the assessment of the quality of the local tie between the telescopes measured by terrestrial surveys.
In this contribution, we address the process of forming single- and double-difference observations between the ranges from two stations to two GNSS satellites, but also the remaining errors sources. We study the suitability of the single-difference strategy to identify and reduce satellite orbit errors and, of special importance, the retro-reflector characteristics and errors for the GNSS satellites, and we investigate the use of double-difference observations for the analysis of station-dependent range biases and calibration errors. We derive accurate estimates for both, the satellite- and station-specific errors, in particular, the possible GNSS retro-reflector biases, demonstrating that systematic effects can be identified at the mm-level. To conclude, the accuracy of the local tie between the two telescopes is assessed through the use of the SLR single- and double-difference observations. The outcomes of these multiple experiments are expected to contribute to the understanding of the biases present in the SLR technique, with the overarching goal of improving the ITRF realization.
The very short baseline formed by the two co-located SLR telescopes (WLRS and SOS-W) at the Geodetic Observatory in Wettzell (Germany) and the quasi-simultaneous tracking of several GNSS satellites provides the opportunity to test these new observation and processing strategies in SLR, enabling the analysis of instrumental biases and the assessment of the quality of the local tie between the telescopes measured by terrestrial surveys.
In this contribution, we address the process of forming single- and double-difference observations between the ranges from two stations to two GNSS satellites, but also the remaining errors sources. We study the suitability of the single-difference strategy to identify and reduce satellite orbit errors and, of special importance, the retro-reflector characteristics and errors for the GNSS satellites, and we investigate the use of double-difference observations for the analysis of station-dependent range biases and calibration errors. We derive accurate estimates for both, the satellite- and station-specific errors, in particular, the possible GNSS retro-reflector biases, demonstrating that systematic effects can be identified at the mm-level. To conclude, the accuracy of the local tie between the two telescopes is assessed through the use of the SLR single- and double-difference observations. The outcomes of these multiple experiments are expected to contribute to the understanding of the biases present in the SLR technique, with the overarching goal of improving the ITRF realization.
Attendee47
Institute Of Geodesy And Geoinformatics
Galileo-based Earth rotation parameters derived with a daily and sub-daily resolution
Abstract Text
Variations in the Earth’s rotation can be examined in the low-frequency and the high-frequency temporal scale. The low-frequency variations are dominated by the annual and Chandler wobbles, while the high-frequency variations are primarily caused by tidal effects and mass redistributions within the system Earth. Depending on the purpose, the Earth Rotation Parameters (ERPs) can be estimated in different time resolutions using space-geodetic techniques, especially using GNSS. However, the residual signals between different techniques or satellite constellations indicate system-specific differences, which have to be properly identified.
Since December 2016 the European GNSS Galileo may be considered as partially operational and since January 2019 as fully operational with 24 healthy satellites in space. Galileo satellites have a different revolution period than GPS satellites, and moreover, two additional Galileo satellites fly on an eccentric orbit which helps to decorrelate tidal constituents from orbit parameters.
This contribution shows the Galileo-based ERPs from a 2-year (2017-2018) global processing. We test different sampling intervals of estimated ERPs from 1h to 24h. The Galileo-based estimates are compared to the results delivered by GPS, GLONASS, and the combined multi-GNSS solution. The sub-daily estimates are also compared with the model from the IERS 2010 Conventions.
In 2017-2018, Galileo delivered the Earth Rotation Parameters of the comparable quality to those from the GPS and of better quality than GLONASS. The RMS of differences between subsequent series and the IERS C04 14 equals 69, 66, 134, 72 µas for the X pole, 57, 57, 123, 66 µas for the Y pole, for the combined, GPS-only, GLONASS-only, and Galileo-only solutions, respectively. Despite that, both GLONASS and Galileo comprise 3 orbital planes, Galileo is not vulnerable to the same spurious signals as GLONASS. Each system shows systematics at different harmonics of the draconitic period. Moreover, an artificial signal with a period close to 3.4 days is visible for the majority of the Galileo-derived parameters. We identify this signal as a consequence of the combination of the Galileo revolution period and the solution length. Eventually, the secular drift of the accumulated UT1-UTC values is 5 times smaller for Galileo than for GPS.
A major issue of the GPS-derived sub-daily ERPs originates from the overlapping periods of the diurnal and semidiurnal tidal terms and the GPS revolution period, which equals half of the sidereal day. The effect of the prograde diurnal signal would remain in case of 1-day GPS orbit, however, in the case of the Galileo solution, the problem significantly decreases due to a much weaker orbital resonance 17:10 with the Earth rotation. Finally, we show the impact of the propagation of signals from a sub-daily ERPs into the estimated GNSS orbits depending on the satellite constellation.
Since December 2016 the European GNSS Galileo may be considered as partially operational and since January 2019 as fully operational with 24 healthy satellites in space. Galileo satellites have a different revolution period than GPS satellites, and moreover, two additional Galileo satellites fly on an eccentric orbit which helps to decorrelate tidal constituents from orbit parameters.
This contribution shows the Galileo-based ERPs from a 2-year (2017-2018) global processing. We test different sampling intervals of estimated ERPs from 1h to 24h. The Galileo-based estimates are compared to the results delivered by GPS, GLONASS, and the combined multi-GNSS solution. The sub-daily estimates are also compared with the model from the IERS 2010 Conventions.
In 2017-2018, Galileo delivered the Earth Rotation Parameters of the comparable quality to those from the GPS and of better quality than GLONASS. The RMS of differences between subsequent series and the IERS C04 14 equals 69, 66, 134, 72 µas for the X pole, 57, 57, 123, 66 µas for the Y pole, for the combined, GPS-only, GLONASS-only, and Galileo-only solutions, respectively. Despite that, both GLONASS and Galileo comprise 3 orbital planes, Galileo is not vulnerable to the same spurious signals as GLONASS. Each system shows systematics at different harmonics of the draconitic period. Moreover, an artificial signal with a period close to 3.4 days is visible for the majority of the Galileo-derived parameters. We identify this signal as a consequence of the combination of the Galileo revolution period and the solution length. Eventually, the secular drift of the accumulated UT1-UTC values is 5 times smaller for Galileo than for GPS.
A major issue of the GPS-derived sub-daily ERPs originates from the overlapping periods of the diurnal and semidiurnal tidal terms and the GPS revolution period, which equals half of the sidereal day. The effect of the prograde diurnal signal would remain in case of 1-day GPS orbit, however, in the case of the Galileo solution, the problem significantly decreases due to a much weaker orbital resonance 17:10 with the Earth rotation. Finally, we show the impact of the propagation of signals from a sub-daily ERPs into the estimated GNSS orbits depending on the satellite constellation.
Attendee99
ETH Zurich
Noise Model of the Galileo “mm-Clock” and the Relativistic Effects
Abstract Text
Galileo is the first GNSS equipped with H-masers. We developed a very accurate noise model of the Passive H-Maser (PHM) used as a primary clock onboard the Galileo navigation satellites. Based on this model we show that estimated Galileo satellite clock parameters completely absorb variations of the radial errors along the orbit and can be used as "continuous SLR" to map radial orbit perturbations continuously along the orbit with smaller standard deviation compared to SLR measurements. We already demonstrated that the noise contribution of the Galileo passive maser on-board IOV satellites has a standard deviation of only 15.5 mm, after removing a linear model (time bias and time drift) from the epoch-wise clock parameters over a typical period of 24 h. In order to decouple the orbit error from the clock noise in the estimated Galileo clock parameters, we consider a period of Galileo data with a high Sun elevation angle (>60°) above the orbit plane of the Galileo satellite. In that case, the orbit errors originating from the modelling of solar radiation pressure and other effects are very modest compared to the rest of the draconitic year of the Galileo orbit. Our analysis is based on the noise simulation of the Galileo PHM clock performance as measured during on-ground tests and the estimated Galileo clock parameters from the MGEX Campaign of IGS, and on an independent validation with SLR. For the clock modelling, we consider all the relevant noise processes, including white frequency noise, flicker frequency noise, white phase noise as well as frequency drift. We considered effects such as the periodic relativistic contribution of the J2 gravity field coefficient as well as the relativistic effects due to the gravity field of the Moon and the Sun, and the environmental effects, such as variations in temperature and magnetic field integrated along the orbit.
In the second part we focus on the relativistic effects for the two Galileo satellites in elliptical orbits based on this very accurate noise model developed for the clocks in the Galileo constellation.
In the second part we focus on the relativistic effects for the two Galileo satellites in elliptical orbits based on this very accurate noise model developed for the clocks in the Galileo constellation.
Attendee28
CNES
Galileo Precise Positioning with Ambiguity Resolution and its contribution to Earth Rotation solutions
Abstract Text
The Centre National d’Etudes Spatiales (CNES) and the Collecte Localisation Satellites (CLS) Group are an Analysis Center of the International GNSS Service (IGS). In October 2018, they announced the provision of high-quality Galileo orbits (~5 cm 3D RMS) and Integer Recovery Clock (IRC) products, using the zero-difference ambiguity resolution method within a multi-GNSS processing with GPS, Galileo and GLONASS (unfixed) constellations. Orbit and clock products are weekly provided to the users, and their performance is tested through 12-hour orbit and clock overlaps, Ambiguity Resolution (AR) success rates, as well as through a new way of integer-value matrix comparison tool.
This method of resolving ambiguities enables one single point (i.e. single RINEX) Precise Point Positioning with Ambiguity Resolution (PPP-AR) solution. Solutions for several combinations of GNSS PPP and PPP-AR are shown and compared. Results are given also for the Galileo-only PPP and PPP-AR solution. Tests include the use of stations within and without the network of stations used by the AC.
Early studies about the contribution of the Galileo system (using several months of Galileo-only products) to the calculation of the Earth Orientation Parameters (EOP), with emphasis to the Pole coordinates and the Length of Day (LOD) are shown by the form of spectral analysis graphs. Galileo-only results are then compared to the GPS-only ones. Finally, conclusions and interpretations of the results are given.
This method of resolving ambiguities enables one single point (i.e. single RINEX) Precise Point Positioning with Ambiguity Resolution (PPP-AR) solution. Solutions for several combinations of GNSS PPP and PPP-AR are shown and compared. Results are given also for the Galileo-only PPP and PPP-AR solution. Tests include the use of stations within and without the network of stations used by the AC.
Early studies about the contribution of the Galileo system (using several months of Galileo-only products) to the calculation of the Earth Orientation Parameters (EOP), with emphasis to the Pole coordinates and the Length of Day (LOD) are shown by the form of spectral analysis graphs. Galileo-only results are then compared to the GPS-only ones. Finally, conclusions and interpretations of the results are given.
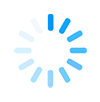