Space Service Volume I
Tracks
Room E4
Thursday, September 5, 2019 |
9:00 AM - 10:40 AM |
Details
Chair: Dr. J. Ventura-Traveset (ESA/ESAC)
Speaker
Attendee86
ESA
Use of GNSS for lunar missions and ESA plans for Lunar IOD
Abstract Text
In the context of Deep Space Exploration as planned by the International Space Exploration Coordination Group (ISECG), the Moon represents a significant step to feed forward what could be done on Mars. The main interests for the lunar exploration are related to In-Situ Resource Utilization (ISRU), tourism, science and mining. The most famous lunar project, the “Moon Village” and the Chinese lunar exploration mission, Chang’e 4, to only cite a few, demonstrate how the interest in uncovering our Moon mysteries continues to gain momentum.
The use of Earth GNSS signals at Moon altitude has been extensively studies in the past, within and outside ESA. This interest has been reflected in the "The Interoperable Global Navigation Satellite Systems Space Service Volume" issued by the International Committee on GNSS (IGC) in 2018. The main limitations has always been the limited knowledge of the actual GNSS antenna patterns outside the main lobe. ESA published the first patterns of GPS satellites using data collected in GIOVE-A and recently NASA shared very detailed 3D patterns of all GPS families. This allows both ESA and NASA to perform detailed analysis of the expected visibility of GNSS signals at moon altitude, in particular NASA published results using GPS only showing that GPS can be received well at Moon altitude (above 22dBHz with 14dBi antenna).
In this presentation we plan to show the results of numerical simulation using GPS and Galileo satellites, in both cases using detailed 3D antenna patterns. The results shows that an high number of satellites can be seen at Moon altitude using a 14dBi antenna and the advantage of Galileo and overall wideband signals for demodulation of the navigation message. The second part of the presentation will provide ESA plans for in orbit demonstration of the use of GNSS at Moon altitude, covering both CubeSat mission and Deep Space Gateway.
The use of Earth GNSS signals at Moon altitude has been extensively studies in the past, within and outside ESA. This interest has been reflected in the "The Interoperable Global Navigation Satellite Systems Space Service Volume" issued by the International Committee on GNSS (IGC) in 2018. The main limitations has always been the limited knowledge of the actual GNSS antenna patterns outside the main lobe. ESA published the first patterns of GPS satellites using data collected in GIOVE-A and recently NASA shared very detailed 3D patterns of all GPS families. This allows both ESA and NASA to perform detailed analysis of the expected visibility of GNSS signals at moon altitude, in particular NASA published results using GPS only showing that GPS can be received well at Moon altitude (above 22dBHz with 14dBi antenna).
In this presentation we plan to show the results of numerical simulation using GPS and Galileo satellites, in both cases using detailed 3D antenna patterns. The results shows that an high number of satellites can be seen at Moon altitude using a 14dBi antenna and the advantage of Galileo and overall wideband signals for demodulation of the navigation message. The second part of the presentation will provide ESA plans for in orbit demonstration of the use of GNSS at Moon altitude, covering both CubeSat mission and Deep Space Gateway.
Attendee40
Fugro Norway AS
Next generation technology for high-accuracy real-time positioning and timing on-board LEO satellites
Abstract Text
The usage of GNSS receivers in LEO orbit has increased dramatically in recent years, both for commercial and scientific purposes, where the knowledge of satellite’s state vector is a requirement for the processing of data from other on-board sensors.
However, the usage of GNSS for high-accuracy navigation in LEO at sub-decimeter level has been restricted so far to near-real-time or post-processing applications only. This is due to the need of downloading the GNSS raw data and its processing in the ground for the estimation of the high-accuracy orbit for the LEO satellite, by correcting GNSS orbit/clock errors.
In order to address these shortcomings, Fugro is developing its new SpaceStar service, which is the adaptation of Precise Point Positioning (PPP) technology for satellites in LEO orbit, allowing to obtain sub-decimeter accuracy in real-time on-board the satellite. PPP provides homogeneous high-accuracy positioning by correcting the GNSS ranging errors affecting the user’s position accuracy, including GNSS orbit/clock errors together with precise observation modelling. SpaceStar leverages in the Fugro’s existing PPP infrastructure for broadcasting precise real-time GNSS orbit/clock corrections to LEO via global L-band Inmarsat satellites.
In this context, a first SpaceStar receiver is being developed as part of ESA’s NAVISP programme, leveraging on state-of-the-art Software-Defined-Radio (SDR) technology and standard off-the-shelf components with flight heritage and cubesat form factor, provided by Gomspace. The GNSS receiver is implemented via Deimos’ FPGA-based GPS and Galileo receiver, and Fugro’s positioning algorithms, adapted for space dynamics together with a software-based L-band demodulator to obtain real-time GNSS orbit/clock from GEO satellites. The GNSS receiver is based on dual-frequency L1/E1 and L5/E5a, which has the advantage of allowing the removal of GNSS ionospheric delays and high GNSS satellites availability thanks to the dual constellation configuration.
The paper will focus on the achievable state vector accuracy with this technology, which is based on epoch-wise PPP-like position/velocity. This has the advantage of reduced on-board software complexity and processing requirements, since no precise orbit or satellite models need to be embedded in the software.
In particular, results using Sentinel-3A will be presented, comparing a PPP solution with the reference orbits computed by Sentinel’s Precise Orbit Determination (POD) service, and a trade-off between kinematic and reduced-dynamic orbit determination will be presented. In addition, the performance of dual-frequency real-time GPS and Galileo L1/E1 and L5/E5a will be presented, in terms of availability, convergence time and position accuracy using Radio Frequency Constellation simulator with representative LEO missions.
However, the usage of GNSS for high-accuracy navigation in LEO at sub-decimeter level has been restricted so far to near-real-time or post-processing applications only. This is due to the need of downloading the GNSS raw data and its processing in the ground for the estimation of the high-accuracy orbit for the LEO satellite, by correcting GNSS orbit/clock errors.
In order to address these shortcomings, Fugro is developing its new SpaceStar service, which is the adaptation of Precise Point Positioning (PPP) technology for satellites in LEO orbit, allowing to obtain sub-decimeter accuracy in real-time on-board the satellite. PPP provides homogeneous high-accuracy positioning by correcting the GNSS ranging errors affecting the user’s position accuracy, including GNSS orbit/clock errors together with precise observation modelling. SpaceStar leverages in the Fugro’s existing PPP infrastructure for broadcasting precise real-time GNSS orbit/clock corrections to LEO via global L-band Inmarsat satellites.
In this context, a first SpaceStar receiver is being developed as part of ESA’s NAVISP programme, leveraging on state-of-the-art Software-Defined-Radio (SDR) technology and standard off-the-shelf components with flight heritage and cubesat form factor, provided by Gomspace. The GNSS receiver is implemented via Deimos’ FPGA-based GPS and Galileo receiver, and Fugro’s positioning algorithms, adapted for space dynamics together with a software-based L-band demodulator to obtain real-time GNSS orbit/clock from GEO satellites. The GNSS receiver is based on dual-frequency L1/E1 and L5/E5a, which has the advantage of allowing the removal of GNSS ionospheric delays and high GNSS satellites availability thanks to the dual constellation configuration.
The paper will focus on the achievable state vector accuracy with this technology, which is based on epoch-wise PPP-like position/velocity. This has the advantage of reduced on-board software complexity and processing requirements, since no precise orbit or satellite models need to be embedded in the software.
In particular, results using Sentinel-3A will be presented, comparing a PPP solution with the reference orbits computed by Sentinel’s Precise Orbit Determination (POD) service, and a trade-off between kinematic and reduced-dynamic orbit determination will be presented. In addition, the performance of dual-frequency real-time GPS and Galileo L1/E1 and L5/E5a will be presented, in terms of availability, convergence time and position accuracy using Radio Frequency Constellation simulator with representative LEO missions.
Attendee23
Deutsches Geoforschungszentrum GFZ
Improvement of GPS Orbit and PCO determination by integrated process with LEO satellites
Abstract Text
The precise orbit determination (POD) of Global Navigation Satellite Systems (GNSS) and Low Earth Orbit satellites (LEOs) are usually performed independently. The GNSS orbits can be improved by integrating LEOs on-board observations in the processing, especially for the emerging GNSS, e.g. Galileo with a sparse ground-sensor-station-network and Beidou with a regional distributed ground network. The integrated processing of ground-based and LEOs (e.g. GRACE-A/B, Jason-2/3, Swarm-A/B/C) onboard code and phase observations is discussed in this study.
In a first study, the POD solutions of the integrated processing with different subsets of the above-mentioned seven LEOs are analyzed in detail. Besides the diversification of LEOs subsets, the rigorously designed ground networks contain different numbers of IGS stations in different geometry. The derived orbits are validated by comparing with official IGS and LEOs-specific products and orbit overlap analysis. Comparing with the GPS-only POD solution derived from a 26-station sparse global ground network, the GPS orbit root mean square (RMS) decreasing from 37.5 mm to 23.9 mm when adding different subsets of LEOs (up to 33.67% improvement). With three LEOs in three different orbital planes integrated, the GPS orbit improves more than the scenario which extends the network with seven additional stations. Both LEOs on-board observation numbers and orbital planes affect the GPS orbit derived by the integrated processing, and the latter one is proved to be more critical. In the processing with a station subset considering Beidou (5 stations in China and 5 stations in other regions), the GPS orbit accuracy improves from about 25cm to 2.5cm after seven LEOs are integrated in the POD.
The GPS phase center offsets and variations (PCO/PCV) providing by IGS via ANTEX are used in the above-mentioned processing. In order to estimate the GPS PCO in addition, a global ground network which contains 56 IGS stations is designed for the processing. The above-mentioned subsets of seven LEOs are also integrated to the estimation. The impact of additional observations and geometry from LEOs on GPS PCO estimation are analyzed in detail. In addition, the need for fixing the network scale by applying the no-net-scale (NNS) constraint in GPS PCO estimation is discussed. For each ground-only or LEOs-integrated scenario, two solutions are derived with and without fixed-scale constraint, respectively. According to the preliminary results, adding LEOs to the estimation reduces the necessity of NNS constraint in GPS PCO estimation.
In a first study, the POD solutions of the integrated processing with different subsets of the above-mentioned seven LEOs are analyzed in detail. Besides the diversification of LEOs subsets, the rigorously designed ground networks contain different numbers of IGS stations in different geometry. The derived orbits are validated by comparing with official IGS and LEOs-specific products and orbit overlap analysis. Comparing with the GPS-only POD solution derived from a 26-station sparse global ground network, the GPS orbit root mean square (RMS) decreasing from 37.5 mm to 23.9 mm when adding different subsets of LEOs (up to 33.67% improvement). With three LEOs in three different orbital planes integrated, the GPS orbit improves more than the scenario which extends the network with seven additional stations. Both LEOs on-board observation numbers and orbital planes affect the GPS orbit derived by the integrated processing, and the latter one is proved to be more critical. In the processing with a station subset considering Beidou (5 stations in China and 5 stations in other regions), the GPS orbit accuracy improves from about 25cm to 2.5cm after seven LEOs are integrated in the POD.
The GPS phase center offsets and variations (PCO/PCV) providing by IGS via ANTEX are used in the above-mentioned processing. In order to estimate the GPS PCO in addition, a global ground network which contains 56 IGS stations is designed for the processing. The above-mentioned subsets of seven LEOs are also integrated to the estimation. The impact of additional observations and geometry from LEOs on GPS PCO estimation are analyzed in detail. In addition, the need for fixing the network scale by applying the no-net-scale (NNS) constraint in GPS PCO estimation is discussed. For each ground-only or LEOs-integrated scenario, two solutions are derived with and without fixed-scale constraint, respectively. According to the preliminary results, adding LEOs to the estimation reduces the necessity of NNS constraint in GPS PCO estimation.
Attendee82
Syderal Swiss
GNSS Space Receiver for On-board Precise Time and Frequency and Signal Regeneration
Abstract Text
LEO mega-constellations are currently developed as telecommunication infrastructures, but they could also be used to provide PNT services, bringing many benefits: propagation loss would be greatly reduced, increasing jamming resistance and enabling indoor usage; higher Doppler would provide enhanced multipath rejection and faster convergence for precise positioning applications, while also enabling Doppler-based positioning; LEO payload manufacturing costs would be lower thanks to economies of scale and a less hostile radiation environment; and the characteristics of the LEO constellation would improve the positioning geometry and make it inherently robust against a single satellite failure. Finally, the same technology could be used to replace the complex and expensive intersatellite link used in other bistatic synthetic aperture radar missions (e.g.: Tandem-x) for timing synchronization.
In this context, a LEO PNT payload is proposed as an augmentation system for an existing GNSS MEO constellation (Galileo). The usage of its signals should be as transparent as possible from the user’s perspective. To achieve this goal, the transmitted signals must be synchronous with Galileo System Time, and precise ephemeris of the LEO satellite shall be broadcasted. Both requirements can be satisfied using a Precise On-board Orbit Determination algorithm, thus removing the costs associated with ground-based orbit and clock error estimation.
This paper describes the LEO PNT EBB project, within which the demonstrator of a spaceborne Galileo receiver able to regenerate a positioning signal perfectly synchronous in time and frequency with the Galileo GNSS signals, is being conceived. The key components of the demonstrator include: a dual-frequency GNSS space receiver prototype; a precise orbit determination algorithm responsible to extract the precise time from the MEO Gaileo signals as well as provide autonomous on-board generation of the LEO ephemeris, thus removing the needs for expensive on-board atomic clock(s) and providing full independence from the ground segment; and the signal generation and transmission chain of the LEO PNT signal being broadcasted towards the LEO PNT user.
In this context, a LEO PNT payload is proposed as an augmentation system for an existing GNSS MEO constellation (Galileo). The usage of its signals should be as transparent as possible from the user’s perspective. To achieve this goal, the transmitted signals must be synchronous with Galileo System Time, and precise ephemeris of the LEO satellite shall be broadcasted. Both requirements can be satisfied using a Precise On-board Orbit Determination algorithm, thus removing the costs associated with ground-based orbit and clock error estimation.
This paper describes the LEO PNT EBB project, within which the demonstrator of a spaceborne Galileo receiver able to regenerate a positioning signal perfectly synchronous in time and frequency with the Galileo GNSS signals, is being conceived. The key components of the demonstrator include: a dual-frequency GNSS space receiver prototype; a precise orbit determination algorithm responsible to extract the precise time from the MEO Gaileo signals as well as provide autonomous on-board generation of the LEO ephemeris, thus removing the needs for expensive on-board atomic clock(s) and providing full independence from the ground segment; and the signal generation and transmission chain of the LEO PNT signal being broadcasted towards the LEO PNT user.
Attendee108
Technical University Of Munich
Phase Biases of GPS and Galileo Signals for the Purpose of Zero-difference Ambiguity Resolution
Abstract Text
Ambiguity resolution of a single receiver requires dedicated satellite orbit, clock, and wide-lane bias products. We estimate GPS wide-lane bias and clock products with respect to C1C, L1C, C2W, L2W. The resulting products are employed into Sentinel-3A/B satellite precise orbit determination. Compared to float solution, the ambiguity-fixed reduced-dynamic solution reduces the standard deviation of satellite laser ranging residuals by about 10%. Furthermore, the ambiguity fixed kinematic solution marks a notable improvement of more than a factor of two over the float kinematic solution by comparing to the reduced-dynamic solution. For Galileo satellites, some receivers track pilot-only signal (L5Q) while some use the cross-correlation technique that reports L5X signal. To assess the difference of these two signals we did initial experiments by setting up individual station clusters for L5Q-only and L5X-only receivers from the MGEX network to estimate the corresponding phase biases. The preliminary results show that the ambiguity fixing rate by using L5Q-only network is in general higher than that by using the L5X-only network. The reason might be the fact that different receiver types employ different weightings in generating the L5X signal. We analyze whether signal specific phase bias is needed. In addition, we also did the joint processing of GPS L1/L5 and Galileo E1/E5a since the frequencies are identical. The results demonstrate that it is beneficial for the Galileo satellites to be processed together with GPS L1/L5 signals. Results concerning Galileo signals based on extended experiments will be presented.
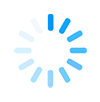