GNSS Science Transversal
Tracks
Room E4
Friday, September 6, 2019 |
11:10 AM - 12:30 PM |
Details
Chair: Prof. H. Kuusniemi (Finnish Geospatial Research Inst.)
Speaker
Attendee132
ESA
Galileo Satellite Metadata for scientific products, source and future updates.
Abstract Text
For scientific GNSS applications such as earthquake monitoring, tsunami early warning, time and frequency transfer or many applications relevant to society as a whole, precise knowledge of the GNSS satellites’ position and clock state is a key element. In order to compute orbits and clocks with one centimeter accuracy and better, GNSS analysts need to have access to the “metadata” related to those satellites.
For GPS and GLONASS, in the framework of the International GNSS Service (IGS), metadata values are not available and are computed empirically what limits the precision of such value as well as hampers the accuracy of scientific products.
Galileo is the first GNSS system providing a full set of these parameters and paving the way for reviewing scientific products with this additional information. The key metadata disclosed for scientific applications comprises:
• Satellite reference frame
• Spacecraft mass and center of mass position and evolution
• Antenna centre of phase and phase centre variation for each signal
• Spacecraft nominal attitude model for body and solar arrays
• Spacecraft solar radiation simplified face model with dimensions of main body and solar panels and materials properties with solar reflectivity, absorption and emission coefficients
• Differential instrumental delays
• Location of laser retro reflector array optical centre.
This list was elaborated in 2011 in the early stage of developments of the Galileo project by the scientific community, the first requested values from IOV were published by the Galileo Service Centre in 2016, updated in 2017 with FOC satellites and completed in 2019. Other GNSS systems followed later Galileo with partial release of the values.
After the Galileo FOC deployment and full metadata disclosure, it is the time to review together with the scientific community the published values, as well as to define, based on scientific needs, future updates from current and future Galileo satellites.
For GPS and GLONASS, in the framework of the International GNSS Service (IGS), metadata values are not available and are computed empirically what limits the precision of such value as well as hampers the accuracy of scientific products.
Galileo is the first GNSS system providing a full set of these parameters and paving the way for reviewing scientific products with this additional information. The key metadata disclosed for scientific applications comprises:
• Satellite reference frame
• Spacecraft mass and center of mass position and evolution
• Antenna centre of phase and phase centre variation for each signal
• Spacecraft nominal attitude model for body and solar arrays
• Spacecraft solar radiation simplified face model with dimensions of main body and solar panels and materials properties with solar reflectivity, absorption and emission coefficients
• Differential instrumental delays
• Location of laser retro reflector array optical centre.
This list was elaborated in 2011 in the early stage of developments of the Galileo project by the scientific community, the first requested values from IOV were published by the Galileo Service Centre in 2016, updated in 2017 with FOC satellites and completed in 2019. Other GNSS systems followed later Galileo with partial release of the values.
After the Galileo FOC deployment and full metadata disclosure, it is the time to review together with the scientific community the published values, as well as to define, based on scientific needs, future updates from current and future Galileo satellites.
Attendee103
The Ohio State University
Deployment of autonomous vehicles in smart cities: primary challenges
Abstract Text
In the next decade, the impact of Smart Cities is likely to be transformative to the way that people live, move, work, and play, and the implications will be felt across geographies, cultures and industries. Smart Cities are those that have a base level of connectivity and integrated municipal services that build on Smart solutions and technology that will lead to the adoption of at least 5 of the 8 following smart parameters: smart energy, smart buildings, smart mobility, smart healthcare, smart infrastructure, smart technology, smart governance and smart education, and smart citizens.
This paper will introduce the definitions of smart city and smart mobility, and the associated technological requirements, with the emphasis on smart mobility. Next, the notion of automated and autonomous driving in a smart city will be discussed, followed by the specifications of requirements on localization, positioning and high definition maps to support autonomous vehicles. High Definition (HD) maps will be defined and their importance to enabling autonomy will be discussed. As vehicle technology is moving towards higher autonomy, the demand for highly accurate map data is rapidly increasing, as accurate and up-to-date maps have a high potential to increase safety. In particular, the quality of the 3D high definition data, measured in accuracy and currency must be clearly far superior to any conventional map data, traditionally provided by federal and local governments. Its contextual information must also be significantly richer than the typical 2D map data used in car navigation systems. In a Smart City environment, integrated data exchange can provide the link between the vehicles and geospatial/GIS database, which includes HD maps and the corresponding metadata. This information is essential to autonomous vehicles as they apply their exteroceptive sensor systems to accurately relate the vehicle’s location to the surrounding environment.
Next, a perspective on testing and validation the accuracy and safety specifications in autonomous vehicles, including the associated challenges, will be offered. Vehicle level testing is still considered insufficient to ensure safety, since safety requirements are inevitably intertwined with the functional performance. Artificial intelligence, heavily used in self-driving cars, learns based on training data, and it may be impossible to separate safety-critical from operational aspects or replicates the training scenarios. Consequently, since the primary challenges of stochastic systems are the use of non-deterministic algorithms (e.g., route planning, perception systems) based on random generation of candidates, they become virtually impossible to reproduce. Similarly, sensor models are based on physics of sensors but their error models include stochastic part. Thus, there is effectively no unique correct behavior for a given test case. Autonomous safety standards and the new testing procedures will be discussed. Examples of proving grounds, such as the Smart Belt Coalition, Ohio Smart Mobility Corridor – a 35-mile stretch of highway between Dublin and East Liberty, Ohio, and the Smart Center, currently under development at The Ohio State University’s Transportation Research Center, will be provided. Finally, conclusions will be offered, with the emphasis on the open questions related to the deployment of autonomous vehicles.
This paper will introduce the definitions of smart city and smart mobility, and the associated technological requirements, with the emphasis on smart mobility. Next, the notion of automated and autonomous driving in a smart city will be discussed, followed by the specifications of requirements on localization, positioning and high definition maps to support autonomous vehicles. High Definition (HD) maps will be defined and their importance to enabling autonomy will be discussed. As vehicle technology is moving towards higher autonomy, the demand for highly accurate map data is rapidly increasing, as accurate and up-to-date maps have a high potential to increase safety. In particular, the quality of the 3D high definition data, measured in accuracy and currency must be clearly far superior to any conventional map data, traditionally provided by federal and local governments. Its contextual information must also be significantly richer than the typical 2D map data used in car navigation systems. In a Smart City environment, integrated data exchange can provide the link between the vehicles and geospatial/GIS database, which includes HD maps and the corresponding metadata. This information is essential to autonomous vehicles as they apply their exteroceptive sensor systems to accurately relate the vehicle’s location to the surrounding environment.
Next, a perspective on testing and validation the accuracy and safety specifications in autonomous vehicles, including the associated challenges, will be offered. Vehicle level testing is still considered insufficient to ensure safety, since safety requirements are inevitably intertwined with the functional performance. Artificial intelligence, heavily used in self-driving cars, learns based on training data, and it may be impossible to separate safety-critical from operational aspects or replicates the training scenarios. Consequently, since the primary challenges of stochastic systems are the use of non-deterministic algorithms (e.g., route planning, perception systems) based on random generation of candidates, they become virtually impossible to reproduce. Similarly, sensor models are based on physics of sensors but their error models include stochastic part. Thus, there is effectively no unique correct behavior for a given test case. Autonomous safety standards and the new testing procedures will be discussed. Examples of proving grounds, such as the Smart Belt Coalition, Ohio Smart Mobility Corridor – a 35-mile stretch of highway between Dublin and East Liberty, Ohio, and the Smart Center, currently under development at The Ohio State University’s Transportation Research Center, will be provided. Finally, conclusions will be offered, with the emphasis on the open questions related to the deployment of autonomous vehicles.
Attendee171
Senior Research Engineer
Septentrio
Assessment of an RTK/INS system for UAV applications
Abstract Text
In recent years Unmanned Aerial Vehicles (UAVs) are developing into an ideal platform for a range of scientific applications, such as earth observation or monitoring. UAVs are becoming the standard in large-scale surveying campaigns, serving as platforms for photogrammetry and LiDAR scanning.
These applications require a GNSS/INS system that provides attitude in addition to timing and position information. The accurate position and timing are needed for the correct geo-referencing of the collected data. For photogrammetry, attitude can aid in stitching the photographs. For LiDAR scanning applications, high data-rate attitude information is needed to correct for platform motion and to remove artifacts from the point cloud.
In recent years, GNSS/INS systems based on MEMS IMUs have become available, fitting the strict weight and power budgets of UAV missions as well as driving down the costs. Some of these systems combine RTK with INS for the demanding UAV surveying applications.
The large amounts of data produced in these applications do not allow for manual corrections of the sensor data after the measurement campaign or after post-processing. The RTK/INS system should be robust and provide reliable and accurate results in real-time or after post-processing. The robustness is needed to limit the downtime and prevent repeating part of the mission. This means the system needs to work every time and in all conditions. The reliability ensures that the solution provided by the system can be trusted which removes the need to manually inspect the data. The accuracy of the system directly influences the accuracy of the final outcome of the UAV mission.
This presentation will describe the assessment of an RTK/INS system, specifically developed for UAV survey and inspection applications. The performance of the RTK/INS system is compared with other commercially available MEMS-based GNSS/INS systems. The systems are mounted together on a large X8 octocopter UAV (28 kg). All systems use dual-antenna GNSS receivers and share the same two antennas, separated by a two-meter baseline for GNSS attitude. The GNSS attitude adds information to the MEMS-based INS filter, which for a multi-copter can improve attitude accuracy during low dynamics (e.g. hovering, straight and level flight) and improves reliability at startup.
The UAV is equipped with a high-grade FARO LiDAR. This provides an indirect quantification of position and attitude accuracy. The LiDAR data collected onboard the UAV is geo-referenced with the position and attitude solutions of each of the GNSS/INS systems under test to generate LiDAR point clouds. In addition, terrestrial data is collected with the same LiDAR mounted on the ground. This provides a millimeter-accurate geo-referenced model of known structures. The performance of each GNSS/INS solution can be assessed by comparing the accuracy of the UAV-based point clouds with respect to the ground-based point cloud on known features. Since the same LiDAR data is used for each of the GNSS/INS systems, this serves as an independent reference to determine the final geo-referencing accuracy of each of the systems.
These applications require a GNSS/INS system that provides attitude in addition to timing and position information. The accurate position and timing are needed for the correct geo-referencing of the collected data. For photogrammetry, attitude can aid in stitching the photographs. For LiDAR scanning applications, high data-rate attitude information is needed to correct for platform motion and to remove artifacts from the point cloud.
In recent years, GNSS/INS systems based on MEMS IMUs have become available, fitting the strict weight and power budgets of UAV missions as well as driving down the costs. Some of these systems combine RTK with INS for the demanding UAV surveying applications.
The large amounts of data produced in these applications do not allow for manual corrections of the sensor data after the measurement campaign or after post-processing. The RTK/INS system should be robust and provide reliable and accurate results in real-time or after post-processing. The robustness is needed to limit the downtime and prevent repeating part of the mission. This means the system needs to work every time and in all conditions. The reliability ensures that the solution provided by the system can be trusted which removes the need to manually inspect the data. The accuracy of the system directly influences the accuracy of the final outcome of the UAV mission.
This presentation will describe the assessment of an RTK/INS system, specifically developed for UAV survey and inspection applications. The performance of the RTK/INS system is compared with other commercially available MEMS-based GNSS/INS systems. The systems are mounted together on a large X8 octocopter UAV (28 kg). All systems use dual-antenna GNSS receivers and share the same two antennas, separated by a two-meter baseline for GNSS attitude. The GNSS attitude adds information to the MEMS-based INS filter, which for a multi-copter can improve attitude accuracy during low dynamics (e.g. hovering, straight and level flight) and improves reliability at startup.
The UAV is equipped with a high-grade FARO LiDAR. This provides an indirect quantification of position and attitude accuracy. The LiDAR data collected onboard the UAV is geo-referenced with the position and attitude solutions of each of the GNSS/INS systems under test to generate LiDAR point clouds. In addition, terrestrial data is collected with the same LiDAR mounted on the ground. This provides a millimeter-accurate geo-referenced model of known structures. The performance of each GNSS/INS solution can be assessed by comparing the accuracy of the UAV-based point clouds with respect to the ground-based point cloud on known features. Since the same LiDAR data is used for each of the GNSS/INS systems, this serves as an independent reference to determine the final geo-referencing accuracy of each of the systems.
Attendee143
University of Alcalá
Penguin movements and ocean dynamics: the role of animal tracking in a changing world
Abstract Text
Biological and physical systems interact over multiple spatial and temporal scales, and integrated knowledge is required to understand a complexity that continues to reshape the earth. A good exponent of such interacting systems comes from seabirds and their dependence on oceans. The open ocean is a heterogeneous environment characterized by a variety of physical features that result in a variable and uncertain distribution of food resources for pelagic predators. As a result, seabirds often need to make large movements in search for food. Monitoring animal movement to understand their spatio-temporal distribution is of paramount importance to identify population trends, potential threats as well as adequate conservation measures. The recent development of miniaturized bio-logging devices has allowed the study of fine-scale distribution and behavior of seabirds while they are travelling, feeding or searching for food at sea. Penguins are among the long-lived seabirds that show foraging dispersal and should adjust their behavior to search for food. In the present study, GPS loggers were used during periods of five days on the chinstrap penguin (Pygoscelis antarctica) in two different years. Penguin movements may also be driven by ocean dynamics; in that case, trajectories could be used as proxies to investigate the dynamics of marine systems and their spatio-temporal evolution. To consider the temporal variability in the ocean state, long-term animal tracking would be needed. However, the difficulty of tracking animal movements over long periods in the pelagic marine environment is still a challenge, moreover under the conditions imposed by the extreme polar environment. Therefore, satellite data is critical for studying the large-scale physical and biological factors that correlate with penguin movements. Such information would provide valuable inputs for the essential activity of monitoring the effects of global change. In that context, we suggest to explore the Europe’s Global Navigation Satellite System of Galileo in penguins, to fully understand how biological and physical processes interact over multiple spatial and temporal scales.
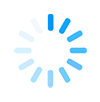