Timing II
Tracks
Room E4
Wednesday, September 4, 2019 |
3:50 PM - 5:30 PM |
Details
Chair: Dr. P. Defraigne (Royal Obs. Belgium)
Speaker
Attendee37
Gmv
PulChron: A new pulsar-based time scale realization
Abstract Text
Coordinated Universal Time (UTC) is now the world’s atomic time system, derived from the International Atomic Time (TAI). Both of these are commonly referred to as ‘paper time scales’, as they are not physically or instantaneously realized. TAI is computed by the Bureau International des Poids et Mesures (BIPM) in Paris using data from an ensemble of atomic clocks located at many National Measurement Institutes (NMIs) and other timing institutes around the world. The use of many clocks and an effective average of the data provides the high stability of TAI.
Leap seconds are inserted into TAI to produce UTC, which has the stability of TAI but is linked to the rotation of the Earth. UTC is evaluated a posteriori by the BIPM and published monthly as offsets from the UTC(k) reference time scales of the NMIs in the Circular T. Today, the different laboratories produce physical representations of UTC, which realize the SI second.
As can be noted, the world’s timekeeping is generated based on the SI definition of the second, and is mainly dependent on commercial atomic clocks and caesium fountains for their realization. Other time scales, autonomously generated, can be built by means of other highly stable elements, such as pulsars and can be useful for the monitoring of the atomic time.
Pulsar measurements, i.e. measurements from heavily dense celestial objects emitting radiation in pulses, can be used to realise both physical and paper time scales.
The objective of the PulChron project is to demonstrate the effectiveness of a pulsar timescale for the generation and monitoring of navigation and time in general. Within the PulChron project, two different timescales based on pulsar measurements are envisioned, one being a real time physical timescale and another one being a “paper timescale”.
In the physical timescale, radiotelescope measurements from pulsars are used to steer an active hydrogen maser to generate the new timescale. While in the paper clock, pulsar measurements are combined together with clocks from different stations and satellites, to realize a timescale which is expected to be the most stable one.
Historically, a timescale built with pulsar measurements have been less stable than the ones built using atomic or optical clocks in the short term, but could be competitive in the very long term. By using a combination of the best pulsar measurements, made available by the European Pulsar Time Array (EPTA) telescopes, the PulChron aims at pushing the limits of stability of the newly generated pulsar timescales.
The PulChron project has been developed in the frame of NAVISP (Navigation Innovation and Support Programme), an ESA programme aiming at fostering innovation in the PNT field while supporting industry and ESA member states interests. In this context, it is considered interesting to demonstrate the implementation of a “real time clock” and a “paper time scale” based on pulsar measurements for PNT monitoring.
Leap seconds are inserted into TAI to produce UTC, which has the stability of TAI but is linked to the rotation of the Earth. UTC is evaluated a posteriori by the BIPM and published monthly as offsets from the UTC(k) reference time scales of the NMIs in the Circular T. Today, the different laboratories produce physical representations of UTC, which realize the SI second.
As can be noted, the world’s timekeeping is generated based on the SI definition of the second, and is mainly dependent on commercial atomic clocks and caesium fountains for their realization. Other time scales, autonomously generated, can be built by means of other highly stable elements, such as pulsars and can be useful for the monitoring of the atomic time.
Pulsar measurements, i.e. measurements from heavily dense celestial objects emitting radiation in pulses, can be used to realise both physical and paper time scales.
The objective of the PulChron project is to demonstrate the effectiveness of a pulsar timescale for the generation and monitoring of navigation and time in general. Within the PulChron project, two different timescales based on pulsar measurements are envisioned, one being a real time physical timescale and another one being a “paper timescale”.
In the physical timescale, radiotelescope measurements from pulsars are used to steer an active hydrogen maser to generate the new timescale. While in the paper clock, pulsar measurements are combined together with clocks from different stations and satellites, to realize a timescale which is expected to be the most stable one.
Historically, a timescale built with pulsar measurements have been less stable than the ones built using atomic or optical clocks in the short term, but could be competitive in the very long term. By using a combination of the best pulsar measurements, made available by the European Pulsar Time Array (EPTA) telescopes, the PulChron aims at pushing the limits of stability of the newly generated pulsar timescales.
The PulChron project has been developed in the frame of NAVISP (Navigation Innovation and Support Programme), an ESA programme aiming at fostering innovation in the PNT field while supporting industry and ESA member states interests. In this context, it is considered interesting to demonstrate the implementation of a “real time clock” and a “paper time scale” based on pulsar measurements for PNT monitoring.
Attendee157
Vsl
Comparing Optical Fibre versus GNSS Time and Frequency Transfer Supporting Galileo Infrastructure
Abstract Text
I. INTRODUCTION
Under the framework of the European Space Agency's European GNSS Evolutions Program (EGEP) ID 104, a project was launched to demonstrate trans-national time and frequency links over optical fiber for GNSS timing infrastructure. If successful, the results of this project will be used 1) to guarantee an accurate synchronization between the two Precise Time Facilities (PTFs) hosted in the Galileo Control Centers and 2) to guarantee the traceability of the Galileo System Time to local realizations of UTC operated by European Laboratories participating in the Time Service Provider (TSP). The selected optical fibre technique for this project is the White Rabbit Precision Time Protocol (WR-PTP).
As a verification, the optical fiber time and frequency transfer was compared against a GPS-based time and frequency transfer link using both code phase and carrier phase observation data from multi-frequency GNSS receivers. This GPS-based time and frequency transfer is a well-established techniques with demonstrated uncertainties.
II. TEST BED
For the experimentation, a test bed was established between the two end-nodes: the Belgian Metrology Service (SMD) in Brussels, Belgium and the ESTEC Radio Navigation Laboratory in Noordwijk, The Netherlands. The test bed consists of: 1) WR switches for the optical fibre time transfer and GNSS receivers for independent verification; and 2) a dedicated optical fibre of about 260 km to connect the two end-nodes.
For the optical fibre link, at each end-node a WR switch, was installed. The WR switch at SMD is the 'grandmaster' and transfers UTC(SMD) to the WR slave switch at ESTEC.
For the GPS-link, at SMD, a DICOM GTR 50 timing receiver is used, and at ESTEC, the GPS receiver is a Septentrio PolaRx5 timing receiver. Both receivers are also connected to the respective UTC realisations.
The internal delays of all equipment were calibrated before installation. The calibration of the fibre delay asymmetry was done during the installation. At both ends of the link, the timescale realization is based on a hydrogen maser.
III. RESULTS
The timescale difference UTC(SMD) - UTC(ESTC) has been determined by a WR time transfer link and a GPS-based time transfer link.
Three different tools were used for analyzing the GPS data. Timescale differences based on code phase measurements were determine from CGGTTS data generated by the RISEGNSS software tool. Frequency difference data based on carrier phase measurements was generated with data processed by gLAB PPP analysis and by BIPM IPPP analysis.
With the exception of some incidents where the WR-link lost its lock, the difference between the WR-PTP and GPS techniques in terms of time is approximately 1 ns and is well within the uncertainty of 3.5 ns (k=1) of the GPS-link which is expected to be the dominant uncertainty contribution. The combined uncertainty of the WR is estimated to be below 0.2 ns (k = 1).
The difference between the WR-link and GPS IPPP-link is stable within ± 0.3 ns for most of the time.
Under the framework of the European Space Agency's European GNSS Evolutions Program (EGEP) ID 104, a project was launched to demonstrate trans-national time and frequency links over optical fiber for GNSS timing infrastructure. If successful, the results of this project will be used 1) to guarantee an accurate synchronization between the two Precise Time Facilities (PTFs) hosted in the Galileo Control Centers and 2) to guarantee the traceability of the Galileo System Time to local realizations of UTC operated by European Laboratories participating in the Time Service Provider (TSP). The selected optical fibre technique for this project is the White Rabbit Precision Time Protocol (WR-PTP).
As a verification, the optical fiber time and frequency transfer was compared against a GPS-based time and frequency transfer link using both code phase and carrier phase observation data from multi-frequency GNSS receivers. This GPS-based time and frequency transfer is a well-established techniques with demonstrated uncertainties.
II. TEST BED
For the experimentation, a test bed was established between the two end-nodes: the Belgian Metrology Service (SMD) in Brussels, Belgium and the ESTEC Radio Navigation Laboratory in Noordwijk, The Netherlands. The test bed consists of: 1) WR switches for the optical fibre time transfer and GNSS receivers for independent verification; and 2) a dedicated optical fibre of about 260 km to connect the two end-nodes.
For the optical fibre link, at each end-node a WR switch, was installed. The WR switch at SMD is the 'grandmaster' and transfers UTC(SMD) to the WR slave switch at ESTEC.
For the GPS-link, at SMD, a DICOM GTR 50 timing receiver is used, and at ESTEC, the GPS receiver is a Septentrio PolaRx5 timing receiver. Both receivers are also connected to the respective UTC realisations.
The internal delays of all equipment were calibrated before installation. The calibration of the fibre delay asymmetry was done during the installation. At both ends of the link, the timescale realization is based on a hydrogen maser.
III. RESULTS
The timescale difference UTC(SMD) - UTC(ESTC) has been determined by a WR time transfer link and a GPS-based time transfer link.
Three different tools were used for analyzing the GPS data. Timescale differences based on code phase measurements were determine from CGGTTS data generated by the RISEGNSS software tool. Frequency difference data based on carrier phase measurements was generated with data processed by gLAB PPP analysis and by BIPM IPPP analysis.
With the exception of some incidents where the WR-link lost its lock, the difference between the WR-PTP and GPS techniques in terms of time is approximately 1 ns and is well within the uncertainty of 3.5 ns (k=1) of the GPS-link which is expected to be the dominant uncertainty contribution. The combined uncertainty of the WR is estimated to be below 0.2 ns (k = 1).
The difference between the WR-link and GPS IPPP-link is stable within ± 0.3 ns for most of the time.
Attendee77
Observatoire De Paris
First Calibration of the UTC TWSTFT Link between LNE-SYRTE and PTB Using a Travelling SDR Receiver
Abstract Text
For more than 15 years, Two-Way Satellite Time and Frequency Transfer (TWSTFT) has been a main technique used continuously and regularly in about 20 laboratories worldwide for the generation of Coordinated Universal Time (UTC) and the results are published monthly in the BIPM KCDB (CCTF-K001.UTC) and the BIPM Circular T. The technique relies on a protocol for transmitting and receiving clock signals via a telecommunication satellite and using carrier frequencies in the Ku band. For this purpose, SAtellite Time and Ranging Equipment (SATRE) modems, developed and marketed by TimeTech GmbH, are being operated in the earth stations of the participating time laboratories. The precision of TWSTFT as observed today is limited by an apparent daily variation pattern (diurnal) in the TWSTFT results. In consequence, calibrations using a mobile TWSTFT station are usually limited by the same effect. Recent developments of Software-Defined Radio (SDR) receivers for TWSTFT have demonstrated superior performance in terms of stability [Metrologia 55 (2018) 685-698], and thus the BIPM processes the SDR measurements of the TWSTFT link between LNE-SYRTE and PTB as a UTC backup link since the end of 2017. However, the accuracy in time remains limited to date because the SDR TWSTFT link is calibrated by alignment with the corresponding SATRE TWSTFT link. In this paper we present the first calibration of an SDR TWSTFT link using a travelling SDR receiver developed in LNE-SYRTE driven by a calibration software developed by LNE-SYRTE in collaboration with TL. This work is aimed to improve the calibration accuracy and thus the uncertainty of operational time links, e.g. to improve the generation of UTC or the Galileo ground segment timing infrastructure. At the colloquium, we will present in detail the calibration method used, the calibration results and associated measurement uncertainties for the SDR TWSTFT link between LNE-SYRTE and PTB.
Attendee33
LNE-SYRTE / Observatoire de Paris
A pirate signal nearby L1-Band jamming GNSS stations in Observatoire de Paris
Abstract Text
LNE-SYRTE in Observatoire de Paris (OP, Paris, France) is operating an ensemble of different GNSS stations which stayed over years close to each other in the ns range. In addition, since the last change of the calibrated delays in GPS receivers (February 2017) and of the reference delay value in the Two-Way Satellite Time and Frequency Transfer (TWSTFT) OP station (March 2017), the offsets between GPS Common-View (CV) and TWSTFT were remaining below 2 ns on the links between UTC(OP) and a selection of remote UTC(k). But at the end of November 2018, a large discrepancy either between the different OP GNSS stations or between GPS CV and TWSTFT, together with an irregular loss of GNSS data, could be observed. Because all OP GNSS stations were not impacted the same way, some local equipment failure was suspected first. But when a spectrum analyzer was connected to one of the GNSS antennas, the cause was easily identified. Just below the GNSS L1-band, a powerful signal more than 35 dB above the GNSS signal level is clearly visible. This signal level is indicating that the unexpected signal is transmitted from the ground nearby OP. But, according to the international frequency table of the International Telecommunication Union (ITU), the frequency band 1535-1559 MHz is formally allocated to satellite telecommunication downlink for institutional users. This is therefore a pirate signal. A formal complaint, raised early January 2019 to the French National Frequency Agency (ANFR), is currently under process. The pirate signal has been changing with time, and we observed different kinds of perturbations on the OP GNSS stations. At the Colloquium, we will provide a comprehensive analysis of the effects of this pirate signal, strongly correlated to the different bandwidth rejections among the GNSS antennas. The last update on the situation in OP will be provided too.
Attendee114
University of Vaasa
Towards resilient GNSS timing in energy distribution networks
Abstract Text
Phasor Measurement Units (PMU) measure current and voltage by amplitude and phase angle in the electricity grid using a common time source for synchronization. PMUs are essential to the development of Network Automatic Protection systems in energy distribution networks, especially for future smart grids . The deployment of PMUs in China and USA is already well underway in response to the energy sector challenges and the rapid deployment of smart grids technology. PMU utilization is growing also in Europe. PMUs are typically deployed across remote locations of power networks in nodes requiring a microsecond level of accuracy. Time synchronization is usually provided by global navigation satellite system (GNSS) receivers and allows synchronized real-time measurements of multiple remote points on the grid. This GNSS-based high-precision time synchronization permits comparing measured values (synchrophasors) from different substations far apart leading to deriving information about the system state and dynamic events such as power swing conditions in order to get improved performance. Since the internal time and frequency references in these PMUs are currently based on GNSS receivers they are therefore experiencing the vulnerabilities inherent to GNSS.
This paper will present results of information gathered from transmission systems operators – an analysis where the largest threats lie and how are operators increasing the robustness and preparedness. First, an overview is given of how PMUs typically currently operate to synchronize the measurements in energy distribution networks. Then, an outlook is given on how transmission operators are handling the PMU vulnerabilities due to threats in GNSS availability, reliability and continuity. The analysis is done utilizing industry interviews, inquiries and literature reviews. Finally, suggestions are given on how time and frequency synchronization with the Galileo navigation system in particular will provide more robustness and resilience in future power grids and ensure secure and uninterrupted energy distribution operation.
This paper will present results of information gathered from transmission systems operators – an analysis where the largest threats lie and how are operators increasing the robustness and preparedness. First, an overview is given of how PMUs typically currently operate to synchronize the measurements in energy distribution networks. Then, an outlook is given on how transmission operators are handling the PMU vulnerabilities due to threats in GNSS availability, reliability and continuity. The analysis is done utilizing industry interviews, inquiries and literature reviews. Finally, suggestions are given on how time and frequency synchronization with the Galileo navigation system in particular will provide more robustness and resilience in future power grids and ensure secure and uninterrupted energy distribution operation.
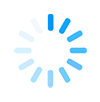