Fundamental Physics I
Tracks
Room E3
Thursday, September 5, 2019 |
9:00 AM - 10:40 AM |
Details
Chair: Dr. P. Delva (SYRTE, Obs. de Paris)
Speaker
Attendee205
University Of Queensland, Australia
Searching for dark matter and exotic physics with atomic clocks in space and on the ground - INVITED
Abstract Text
Despite the overwhelming cosmological evidence for the existence of dark matter, and the considerable effort of the scientific community over decades, there is no evidence for dark matter in terrestrial experiments.
One possibility is that dark matter is made of ultra-light quantum fields, which may act coherently over laboratory length-scales.
As such fields pass by the earth, it may be possible to detect them in precision laboratory experiments.
I will discuss the current efforts and future prospects for searching for dark matter using ground and space-based atomic clocks, some of the most precise instruments ever created, including turning the GPS constellation into a 50,000 km aperture dark matter "telescope" making it the largest particle-physics detector in the world.
One possibility is that dark matter is made of ultra-light quantum fields, which may act coherently over laboratory length-scales.
As such fields pass by the earth, it may be possible to detect them in precision laboratory experiments.
I will discuss the current efforts and future prospects for searching for dark matter using ground and space-based atomic clocks, some of the most precise instruments ever created, including turning the GPS constellation into a 50,000 km aperture dark matter "telescope" making it the largest particle-physics detector in the world.
Attendee107
Royal Observatory Of Belgium
Fundamental physics tests using the propagation of GNSS signals
Abstract Text
New tests of fundamental physics can be set up by looking at perturbations of the GNSS signals propagation. This paper proposes an original theoretical approach and a first quantification of the expected perturbations induced by a specific case of dark matter signature, the domain walls.
System-free expressions of Maxwell’s equations have been initially introduced [1,2] by means of four empirical constants that aim to be independent of any system of units (SI, CGS...). Such unusual expressions of electromagnetic waves propagation allow us to check variations of these constants as a test of fundamental physics. In particular, in the macroscopic Maxwell’s equations, these constants describe the properties of the medium in which the electromagnetic waves propagate. We will thus show that at a macroscopic scale, a variation of these constants would affect the propagation of the signal like an inhomogeneous medium of which the properties are related to the underlying model of new physics.
Some models of dark matter predict the existence of large structures which could regularly cross the Earth trajectory, possibly disturbing GNSS. While the detection of dark matter signatures was already studied from satellite clock behavior [3], we propose here to look additionally into the GNSS signal perturbations. Our method will be illustrated in the case of models for planar structures called domain walls, with identical astrophysical parameters as in [4].
Our results reveal that the signals propagate inside the domain wall as an evanescent wave. The amplitude of the electric field is increased while the intensity remains unaffected. The other impact consists in a phase shift of the GNSS signal’s carrier. By analogy with the atmospheric effects, its origin relies on both the bending of the signal trajectory and the variation of phase velocity. We assess the time delay to 100ps for realistic models of domain walls of which the transverse size is at the scale of the Earth. Such a signature is detectable by correlated observations with several GNSS satellites and stations.
More generally, the study of the propagation of the signals should bring less stringent constraints than techniques based on atomic clocks [4]. However, the analysis of GNSS signals has a larger sensitivity to the size of domain walls ranging from a few centimetres to the solar system scale, and the multi-frequency analysis should bring a useful tool as the effect on the signals depends strongly on the frequency.
[1 ] J. D. Jackson, “Classical Electrodynamics,” John Wiley & Sons, 3rd Edition, New Work, 1998.
[2] P. Leung, “A Note on the System-free Expression of Maxwell’s Equations,” European Journal of Physics, vol. 25, pp. N1-N4, 2004.
[3] A. Derevianko, and M. Pospelov, “Hunting for Topological Dark Matter with Atomic Clocks,” Nature Phys., vol. 10, p.933, 2014.
[4] B. M. Roberts et al., “Search for Domain Wall Dark Matter with Atomic Clocks on Board Global Positioning System Satellites,” Nature Com., vol. 8, 2017.
System-free expressions of Maxwell’s equations have been initially introduced [1,2] by means of four empirical constants that aim to be independent of any system of units (SI, CGS...). Such unusual expressions of electromagnetic waves propagation allow us to check variations of these constants as a test of fundamental physics. In particular, in the macroscopic Maxwell’s equations, these constants describe the properties of the medium in which the electromagnetic waves propagate. We will thus show that at a macroscopic scale, a variation of these constants would affect the propagation of the signal like an inhomogeneous medium of which the properties are related to the underlying model of new physics.
Some models of dark matter predict the existence of large structures which could regularly cross the Earth trajectory, possibly disturbing GNSS. While the detection of dark matter signatures was already studied from satellite clock behavior [3], we propose here to look additionally into the GNSS signal perturbations. Our method will be illustrated in the case of models for planar structures called domain walls, with identical astrophysical parameters as in [4].
Our results reveal that the signals propagate inside the domain wall as an evanescent wave. The amplitude of the electric field is increased while the intensity remains unaffected. The other impact consists in a phase shift of the GNSS signal’s carrier. By analogy with the atmospheric effects, its origin relies on both the bending of the signal trajectory and the variation of phase velocity. We assess the time delay to 100ps for realistic models of domain walls of which the transverse size is at the scale of the Earth. Such a signature is detectable by correlated observations with several GNSS satellites and stations.
More generally, the study of the propagation of the signals should bring less stringent constraints than techniques based on atomic clocks [4]. However, the analysis of GNSS signals has a larger sensitivity to the size of domain walls ranging from a few centimetres to the solar system scale, and the multi-frequency analysis should bring a useful tool as the effect on the signals depends strongly on the frequency.
[1 ] J. D. Jackson, “Classical Electrodynamics,” John Wiley & Sons, 3rd Edition, New Work, 1998.
[2] P. Leung, “A Note on the System-free Expression of Maxwell’s Equations,” European Journal of Physics, vol. 25, pp. N1-N4, 2004.
[3] A. Derevianko, and M. Pospelov, “Hunting for Topological Dark Matter with Atomic Clocks,” Nature Phys., vol. 10, p.933, 2014.
[4] B. M. Roberts et al., “Search for Domain Wall Dark Matter with Atomic Clocks on Board Global Positioning System Satellites,” Nature Com., vol. 8, 2017.
Attendee43
Agenzia Spaziale Italiana
Dark Matter Search by GALILEO
Abstract Text
Dark Matter (D-M) makes up about 85% of all matter in the universe and till now nothing is known about its nature. There is a growing interest in finding alternative candidates to Weakly Interactive Mass Particles (WIMP). Some models provide D-M in the form of spatially large objects that can cause anomalies in atomic clocks such as: Topologic Defects (TD), Q-Balls, or Solitons. TD in turn can be distinguished in Domain Wall (2D), string (1 D) and monopoles (0D). TD may have formed during the cooling of the early universe thanks to a spontaneous transition of the symmetry breaking phase. The flying network of atomic satellites on board of GNSS satellites such as GPS and GALILEO, can be considered ideal detector suitable for finding large bodies of DM, with baselines up to 60,000 km long. If we suppose an isotropic distribution of DM in a resting galactic reference system, the constellation of the GNSS satellites would sweep the DM at a speed of ≈300 km / s ; i.e. the speed of our solar system rotating around the center of the Galaxy. The incoming signal is in principle easily recognizable because its signature consists of a correlated propagation of the anomalies of the clocks of each satellite, lasting a few minutes, when the DM crosses the constellation. The wide geometric range of GNSS network is ideal to look for such a signal. This investigation has already been attempted using a historical series of GPS clock solutions 16 years long with e level of precision of 0.1 nano-sec with no evidence of D-M transits. The Cs and RAFS are the clocks on GPS. We would repeat the search by using GALILEO as well. The combination of GPS+GALILEO provide a formidable flying cluster of Cs, RAFS and PHM clocks. We are confident to replicate the same measurement with few orders of magnitude better at least than the previous experiments, thanks to the special properties of PHM GALILEO clocks. So We will describe the observation strategy and apply tailored signal processing algorithms helpful to extract possible D-M signatures.
Attendee165
National Institute For Astrophysics (inaf)
The Galileo for Science (G4S_2.0) project: planning of the activities for the relativistic measurements and preliminary results
Abstract Text
The G4S_2.0 project aims to perform a set of relativistic measurements with the two Galileo satellites GSAT-5 and GSAT-6 (DORESA and MILENA) exploiting their relatively high eccentricity (about 0.16), a deeper consideration of the satellites tracking data based on the Satellite Laser Ranging (SLR) technique and, finally, the possibility to handle the subtle effects produced by the Non-Gravitational Perturbations (NGP) by the development of a refined Finite Element Model (FEM) for their complex surface. Indeed, from the accurate analysis of the orbits and clocks of these satellites an ensemble of relativistic tests may be performed with the goals to compare the predictions of the gravitational theories regarding, mainly, the motion of a “test” particle along a geodesic of space-time and the time dilation of the onboard clocks. Within the G4S_2.0 project we also proposed to test an inverse relativistic positioning system. Indeed, since light, or more in general an electromagnetic wave, constitutes an intrinsically relativistic probe for investigating space-time, the counting of the pulses from an appropriate set of emitters on-ground may be used to provide null emission coordinates for the receiver onboard a satellite. Consequently, the measurement of proper time intervals between successive arrivals of the pulses from the emitters is used to localize the receiver, within a precision controlled by the onboard clock. In addition to these main aspects, G4S_2.0 aims also to develop two activities that could find an application within next generation of the Galileo satellites. The first activity is finalized to the conception of an accelerometer to be installed on-board the Galileo with the main objective to measure the non-gravitational accelerations directly. The second activity is finalized to exploit the inter-satellite link among the next Galileo satellites in such a way to measure the gravitomagnetic field of the Earth by means of the Sagnac effect on a closed loop constituted by at least three satellites. In this paper we present the state of the art of the preliminary activities we started in 2019. These activities concern modelling problems, precise orbit determination and the different procedures to be exploited for the expected relativistic measurements.
Attendee136
Inaf/oato
Relativistic Positioning and Sagnac-like measurements for fundamental physics in space
Abstract Text
Constellations of terrestrial satellites lend important opportunities for application and for basic research in space. Considering positioning and circumterrestrial navigation, we start from the remark that space-time is a geometric four-dimensional Riemannian manifold. The problem of positioning in space and time in such manifold can be approached in a way similar to topography in two or three dimensions. One starts from a set of four (as the dimensions of the manifold) stations, whose positions in space-time form a reference basis. Thinking of four satellites along the same orbit, we have a “rigid” configuration in space; equipping each station with a clock, we are able to “draw” the world-lines of our beacons. If each satellite emits regular pulses, a traveller receiving them, recording the arrival time sequences, is enabled, by the use of a simple algorithm, to reconstruct its coordinates with respect to the basis. The “drawing” of the world-line of the receiver incorporates the curvature of the manifold, i.e. the effect of gravity. When the satellites, besides emitting regular signals, can also receive them, accurately measuring the arrival times they can evidence an extremely weak effect of general relativity, i.e. gravito-magnetism. The method relies on the generalized Sagnac effect. Consider a polygon (e.g. a triangle) of satellites on the same orbit, then let one of them send electromagnetic signals along the polygon both clock- and anti-clockwise. The other satellites at the vertices of the polygon must act as mirrors or transponders so that each signal, completing a round trip, comes back to the original emitter. In the reference frame of the polygon the path of the signals is closed in space. If all this happens around a massive rotating body (the Earth) the times of flight in opposite directions are different and the difference is proportional to the angular momentum of the central body. This is an effect of the gravito-magnetic field of the Earth. The difference along one loop, for the Galileo satellites, would be in the order of 10-¹⁶ s, which is a very small time interval, but could in principle be measured (for instance cumulating many turns). However, the Earth is not the only source of gravito-magnetism. It is currently accepted that our galaxy is immersed in a dark matter halo, many times more massive than the visible stars. If so it is reasonable to expect that the dark halo revolves more or less at the same rate as visible matter does, which means that it is also endowed with a very big angular momentum, so being a source of gravito-magnetism. If so, our closed loop of Galileo satellites, used à la Sagnac, would sense also the galactic dark angular momentum. If then our triangle is made of two satellites in one orbital plane and one in another, the area of the triangle as well as its orientation with respect to the axis of the Milky Way will be modulated in time, thus marking the galactic dark signal, allowing to distinguish it from the terrestrial contribution.
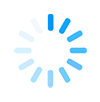