Session 7A: Spacecraft Radiometric Techniques
Tracks
H-I
Thursday, September 26, 2019 |
11:40 AM - 1:00 PM |
Speaker
Attendee81
Thales Alenia Space
JUICE Ka-band Transponder
11:40 AM - 12:00 PMAbstract Submission
This paper presents the Ka-Band Transponder (KaT) for Radioscience that has been designed by Thales Alenia Space Italia (TAS-I) for JUICE mission.
The JUICE KaT is largely recurring from BepiColombo MORE (Mercury Orbiter Radioscience Experiment) KaT, which is the evolution of the first digital Ka-Band translator developed for NASA JUNO mission and the fully analogue K-Band Translator for Cassini mission.
For JUICE mission the KaT has been improved with higher performances in terms of radiation robustness, keeping the same excellent performances in terms of ranging measurements as accurate as 20 cm (two-way) by means of a two-way coherent link at Ka-band from/to an Earth Deep Space Antenna.
The KaT implements the same transponder technology developed and used for the MORE BepiColombo Ka-band Radio Science payload, based on the KaT ASIC, the system-on-chip for TT&C application developed by Thales Alenia Space Italia (TAS-I) in ATMEL ATC18RHA 0.18um technology in the frame of BepiColombo program.
Using an innovative digital-platform concept, the KaT implements a Pseudo-Noise (PN) Ranging modulation scheme up to 24 Mcps. The regenerative ranging scheme is based on pseudo-noise sequence that can be easily regenerated on-board. The net result of the regeneration is an increase in the effective return ranging power. This increase provides some margin for decreasing ranging signal integration time at the ground station, decreasing the power of the return ranging signal transmitted by the transponder or improving the end-to-end ranging accuracy.
PN binary sequences are used because they offer a desirable combination of high ranging resolution, low ranging ambiguity and no need for receivers to “know” when pseudo-noise sequences started as for the sequential ranging. As an alternative to regenerative ranging, the KaT supports also a Transparent ranging channel in order to cope with existing Sequential Ranging Systems such as the ESA Code Ranging System and the NASA Tone Ranging System. The transparent ranging bandwidth achieved is about 27 MHz. The KaT supports an additional ranging scheme that represents a synthesis among regenerative and transparent worlds. Named “Mixed Ranging”, his typical spectrum envelope presents a reduced transparent low-frequency component and a Regenerated ranging tone at 12.1 MHz. In case the Ground-Station could transmit the additional tone, the Mixed Ranging foresees a Tone Recovery Loop for on-board regeneration.
The KaT architecture shows excellent performance in terms of frequency stability, a crucial specification for such kind of Radio Science Instrument. A typical Allan Deviation contribution is less than 10-15 at 1000 s integration time.
The paper will present the architecture, the frequency plan, the Digital Signal Processing capabilities, the mechanical configuration and the measured excellent results achieved in the frame of the first Engineering Model test campaign.
The JUICE KaT is largely recurring from BepiColombo MORE (Mercury Orbiter Radioscience Experiment) KaT, which is the evolution of the first digital Ka-Band translator developed for NASA JUNO mission and the fully analogue K-Band Translator for Cassini mission.
For JUICE mission the KaT has been improved with higher performances in terms of radiation robustness, keeping the same excellent performances in terms of ranging measurements as accurate as 20 cm (two-way) by means of a two-way coherent link at Ka-band from/to an Earth Deep Space Antenna.
The KaT implements the same transponder technology developed and used for the MORE BepiColombo Ka-band Radio Science payload, based on the KaT ASIC, the system-on-chip for TT&C application developed by Thales Alenia Space Italia (TAS-I) in ATMEL ATC18RHA 0.18um technology in the frame of BepiColombo program.
Using an innovative digital-platform concept, the KaT implements a Pseudo-Noise (PN) Ranging modulation scheme up to 24 Mcps. The regenerative ranging scheme is based on pseudo-noise sequence that can be easily regenerated on-board. The net result of the regeneration is an increase in the effective return ranging power. This increase provides some margin for decreasing ranging signal integration time at the ground station, decreasing the power of the return ranging signal transmitted by the transponder or improving the end-to-end ranging accuracy.
PN binary sequences are used because they offer a desirable combination of high ranging resolution, low ranging ambiguity and no need for receivers to “know” when pseudo-noise sequences started as for the sequential ranging. As an alternative to regenerative ranging, the KaT supports also a Transparent ranging channel in order to cope with existing Sequential Ranging Systems such as the ESA Code Ranging System and the NASA Tone Ranging System. The transparent ranging bandwidth achieved is about 27 MHz. The KaT supports an additional ranging scheme that represents a synthesis among regenerative and transparent worlds. Named “Mixed Ranging”, his typical spectrum envelope presents a reduced transparent low-frequency component and a Regenerated ranging tone at 12.1 MHz. In case the Ground-Station could transmit the additional tone, the Mixed Ranging foresees a Tone Recovery Loop for on-board regeneration.
The KaT architecture shows excellent performance in terms of frequency stability, a crucial specification for such kind of Radio Science Instrument. A typical Allan Deviation contribution is less than 10-15 at 1000 s integration time.
The paper will present the architecture, the frequency plan, the Digital Signal Processing capabilities, the mechanical configuration and the measured excellent results achieved in the frame of the first Engineering Model test campaign.
Attendee83
Università di Bologna
Delta-DOR measurements using VLBI antennas
12:00 PM - 12:20 PMAbstract Submission
Deep space missions have critical requirements in terms of orbit determination, so that an accurate knowledge of the spacecraft position is of fundamental importance in order to achieve its success. Information about the position and velocity of the spacecraft is obtained by exploiting radiometric techniques, such as ranging, Doppler and Delta-DOR (Delta-Differential One-way Ranging), giving access to the knowledge of probe distance and relative velocity with respect to the ground antenna and its angular position in the plane of sky respectively.
Delta-DOR is generally formed by multiple DOR observables. The DOR observable, expressed in terms of a time delay, is extracted as the difference between times of arrival of the same signal at two receiving stations, that form a baseline, which is as large as possible in order to increase the spatial accuracy. The differential time delay of the received signal contains information about the angular position with respect to the baseline connecting the two stations. However, such observable is normally affected by errors related to the atmosphere, clock offsets and instrumental electronics.
Therefore, the DOR observable is calibrated by analyzing the signal of a Quasar, which is an extremely far source of electromagnetic white noise: its position is very well known so that its measured time delay contains most of the errors that can be calibrated subtracting the Quasar DOR from the spacecraft DOR, obtaining a Delta-DOR observable.
Nowadays, the Delta-DOR technique is performed by only a few receiving ground stations, due to the particular hardware requirements. In case of ESA missions, the dedicated ESA antennas are three, located in New Norcia (Western Australia), Cebreros (Spain) and Malargüe (Argentina), belonging to the ESTRACK (European Space Tracking) network. The spectrums of the signals are recorded in open loop by equipment capable of storing digital data in RDEF (Raw Data Exchange Format) format. These recordings, together with flight dynamics data, are then processed by a software correlator at ESOC, which finally outputs the desired observable.
The described technique is particularly demanding in terms of resources, requiring two antennas at the same time for this task; this is why looking for possible alternative candidates as receiving antennas is interesting, both in terms of time efficiency and better accuracy, which is achieved by adding more baselines to the problem.
The requirements for this technique are large antenna dishes with cryogenic cooled receivers (characterized by high SNR values), with back-end receivers capable of handling the classical Deep Space mission (Category B) frequency ranges: these hardware requirements are satisfied by the antennas belonging to the VLBI (Very Long Baseline Interferometry) network. The numerous antennas of the VLBI community could then be used for this purpose. However they lack the required RDEF software output, since the processing equipment is capable to store data only in the standard file type of the VLBI, called VDIF (VLBI Data Interchange Format).
In the study described in this paper, a software translator (from VDIF to RDEF) has been designed and implemented, so to allow the software correlator to use the VLBI recordings as an input for extracting the Delta-DOR observable.
To check if the work-chain containing VLBI antennas could be used for Delta-DOR analysis, a shadow pass of a classical Delta-DOR ESA session of the GAIA satellite has been scheduled, considering the baseline New Norcia – Cebreros in November 2018. The Italian VLBI antenna located at Medicina was passively inserted in the tracking session in order to use its output to be correlated with the recordings of the New Norcia ground station.
The recordings of Medicina were stored as VDIF files; they were successively translated to RDEF format and processed by ESA’s software correlator using the Medicina – New Norcia baseline. The output of this correlation process produced results fully compatible with those measured by the standard ESA baseline formed by the Cebreros – New Norcia antennas. This positive result indicates that the entire data acquisition, pre-processing, translation and correlation procedure was carried out correctly. This is the very first time that a VLBI station was used in conjunction with a standard Deep Space tracking antenna to carry out Delta-DOR measurements. Therefore, our initial goal of exploiting VLBI antennas for Delta-DOR analysis has been reached and an experimental proof-of-concept was successfully carried out.
Delta-DOR is generally formed by multiple DOR observables. The DOR observable, expressed in terms of a time delay, is extracted as the difference between times of arrival of the same signal at two receiving stations, that form a baseline, which is as large as possible in order to increase the spatial accuracy. The differential time delay of the received signal contains information about the angular position with respect to the baseline connecting the two stations. However, such observable is normally affected by errors related to the atmosphere, clock offsets and instrumental electronics.
Therefore, the DOR observable is calibrated by analyzing the signal of a Quasar, which is an extremely far source of electromagnetic white noise: its position is very well known so that its measured time delay contains most of the errors that can be calibrated subtracting the Quasar DOR from the spacecraft DOR, obtaining a Delta-DOR observable.
Nowadays, the Delta-DOR technique is performed by only a few receiving ground stations, due to the particular hardware requirements. In case of ESA missions, the dedicated ESA antennas are three, located in New Norcia (Western Australia), Cebreros (Spain) and Malargüe (Argentina), belonging to the ESTRACK (European Space Tracking) network. The spectrums of the signals are recorded in open loop by equipment capable of storing digital data in RDEF (Raw Data Exchange Format) format. These recordings, together with flight dynamics data, are then processed by a software correlator at ESOC, which finally outputs the desired observable.
The described technique is particularly demanding in terms of resources, requiring two antennas at the same time for this task; this is why looking for possible alternative candidates as receiving antennas is interesting, both in terms of time efficiency and better accuracy, which is achieved by adding more baselines to the problem.
The requirements for this technique are large antenna dishes with cryogenic cooled receivers (characterized by high SNR values), with back-end receivers capable of handling the classical Deep Space mission (Category B) frequency ranges: these hardware requirements are satisfied by the antennas belonging to the VLBI (Very Long Baseline Interferometry) network. The numerous antennas of the VLBI community could then be used for this purpose. However they lack the required RDEF software output, since the processing equipment is capable to store data only in the standard file type of the VLBI, called VDIF (VLBI Data Interchange Format).
In the study described in this paper, a software translator (from VDIF to RDEF) has been designed and implemented, so to allow the software correlator to use the VLBI recordings as an input for extracting the Delta-DOR observable.
To check if the work-chain containing VLBI antennas could be used for Delta-DOR analysis, a shadow pass of a classical Delta-DOR ESA session of the GAIA satellite has been scheduled, considering the baseline New Norcia – Cebreros in November 2018. The Italian VLBI antenna located at Medicina was passively inserted in the tracking session in order to use its output to be correlated with the recordings of the New Norcia ground station.
The recordings of Medicina were stored as VDIF files; they were successively translated to RDEF format and processed by ESA’s software correlator using the Medicina – New Norcia baseline. The output of this correlation process produced results fully compatible with those measured by the standard ESA baseline formed by the Cebreros – New Norcia antennas. This positive result indicates that the entire data acquisition, pre-processing, translation and correlation procedure was carried out correctly. This is the very first time that a VLBI station was used in conjunction with a standard Deep Space tracking antenna to carry out Delta-DOR measurements. Therefore, our initial goal of exploiting VLBI antennas for Delta-DOR analysis has been reached and an experimental proof-of-concept was successfully carried out.
Attendee307
Università La Sapienza
Results from the initial tests of the Mercury Orbiter Radioscience Experiment (MORE) on BepiColombo
12:20 PM - 12:40 PMAbstract Submission
The ESA-JAXA spacecraft BepiColombo, launched on 20 October 2018 from Kourou (French Guyana) is currently in cruise to Mercury, its final destination. Its advanced radio system entails a TT&C subsystem enabled by a deep space transponder (DST) supporting a X band uplink (7.2 GHz) and a dual frequency, coherent X and Ka band downlink /8.4 and 32.5 GHz). The ranging function is based on a 3 Mcps pseudo-noise modulation on all channels. The onboard radio system is completed by a separate, coherent link at Ka band (both uplink and downlink) enabled by a Ka band transponder (KaT), built by Thales Alenia Space Italia and part of the Mercury Orbiter Radio science Experiment (MORE). The MORE ranging function uses a 24 Mcps pseudo-noise modulation and exploits also an internal calibration of the KaT range delay. The MORE KaT has been specified for an Allan deviation of 10-15 @ 1000 s integration time and tested at 4x10-16 at the same integration time.
The MORE investigation onboard the Mercury Planetary Orbiter (MPO) aims at determining the gravity field and rotational state (obliquity and physical librations) of the planet. The tracking of Mercury’s orbital motion will provide precise tests of general relativity. Doppler and range measurements collected during the cruise phase will also be used to measure the time delay and frequency shift of the radio signal during superior solar conjunctions, therefore probing the space components of the solar metric.
In May and August 2019 the MORE radio system underwent an initial test phase. The spacecraft was tracked by the ESA DSA-3 antenna in Malargue, Argentina, and NASA’s DSN station DSS 25, located in Goldstone, California, when the spacecraft was at an Earth distance of 0.3-0.5 AU. We report on the first analysis of range and Doppler data. The results generally exceed the expectations, especially for the novel ranging system at Ka band. We show that the 24 Mcps PN modulation, enabled by the MORE KaT and novel hardware at the DSA-3 antenna, provided two-way range measurements as accurate as 7 mm with just 4 s integration time.
The MORE investigation onboard the Mercury Planetary Orbiter (MPO) aims at determining the gravity field and rotational state (obliquity and physical librations) of the planet. The tracking of Mercury’s orbital motion will provide precise tests of general relativity. Doppler and range measurements collected during the cruise phase will also be used to measure the time delay and frequency shift of the radio signal during superior solar conjunctions, therefore probing the space components of the solar metric.
In May and August 2019 the MORE radio system underwent an initial test phase. The spacecraft was tracked by the ESA DSA-3 antenna in Malargue, Argentina, and NASA’s DSN station DSS 25, located in Goldstone, California, when the spacecraft was at an Earth distance of 0.3-0.5 AU. We report on the first analysis of range and Doppler data. The results generally exceed the expectations, especially for the novel ranging system at Ka band. We show that the 24 Mcps PN modulation, enabled by the MORE KaT and novel hardware at the DSA-3 antenna, provided two-way range measurements as accurate as 7 mm with just 4 s integration time.
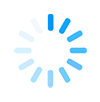