Session 4B: Microsatellites-Cubesats
Tracks
H-IV
Wednesday, September 25, 2019 |
11:40 AM - 1:00 PM |
Speaker
Attendee169
NASA
The Evolution of the Jet Propulsion Laboratory/NASA Iris Deep-Space Transponder
11:40 AM - 12:00 PMAbstract Submission
In 2013, the Jet Propulsion Laboratory (JPL) developed the Iris Deep Space transponder, a CubeSat compatible software-defined radio (SDR), intended to support of the first CubeSat deep space mission: the Interplanetary NanoSpacecraft Pathfinder In Relevant Environment (INSPIRE). The Iris Transponder is a reconfigurable SDR designed for missions requiring interoperability with NASA's Deep Space Network (DSN) on X-band frequencies (7.2 GHz uplink, 8.4 GHz downlink). The transponder provides radiometric tracking support with the DSN to provide navigational products for precise orbit determination while performing standard uplink and downlink communications in a CubeSat/SmallSat-applicable package size.
In 2015, JPL developed the second version of the Iris Deep-Space Transponder to be used on the Mars Cube One (MarCO) mission. The second version has in addition to X-Band transmit and receive functionality, the capability to receive in the UHF band. For MarCO, Iris successfully performed bent-pipe relay direct-to-Earth during entry, descent, and landing (EDL) of the InSight lander, providing the first confirmation of successful landing, including InSight’s first image from the surface of Mars.
Currently, a third version of Iris is being developed to be used on secondary payload missions of the upcoming Space Launch System Exploration Mission One (SLS EM-1). In this version, several updates were made. The power supply board (PSB) was redesigned to increase the radiation tolerance, and further miniaturization was performed to reduce the overall SwaP (Size, Weight, and Power) of the unit. Also, two enhanced navigation and ranging techniques were added. Namely, the Pseudo-random Noise (PN) Delta-Difference-of-Ranging (DDOR) technique as well as PN Regenerative Ranging.
This presentation/paper discusses the evolution of the Iris transponder and provides a summary of the key design aspects and specifications and presents some results from lab testing as well as flight behavior for the MarCO-Iris case.
In 2015, JPL developed the second version of the Iris Deep-Space Transponder to be used on the Mars Cube One (MarCO) mission. The second version has in addition to X-Band transmit and receive functionality, the capability to receive in the UHF band. For MarCO, Iris successfully performed bent-pipe relay direct-to-Earth during entry, descent, and landing (EDL) of the InSight lander, providing the first confirmation of successful landing, including InSight’s first image from the surface of Mars.
Currently, a third version of Iris is being developed to be used on secondary payload missions of the upcoming Space Launch System Exploration Mission One (SLS EM-1). In this version, several updates were made. The power supply board (PSB) was redesigned to increase the radiation tolerance, and further miniaturization was performed to reduce the overall SwaP (Size, Weight, and Power) of the unit. Also, two enhanced navigation and ranging techniques were added. Namely, the Pseudo-random Noise (PN) Delta-Difference-of-Ranging (DDOR) technique as well as PN Regenerative Ranging.
This presentation/paper discusses the evolution of the Iris transponder and provides a summary of the key design aspects and specifications and presents some results from lab testing as well as flight behavior for the MarCO-Iris case.
Attendee94
Serco Nederland Bv for ESA
A procedure to recover data of a cubesat mission at very low S/N ratio
12:00 PM - 12:20 PMAbstract Submission
Cubesat missions success is often negatively affected by a reduced amount of available telemetry data with respect to expectations. One of the most common causes is the difficulty of signal reception, demodulation and decoding due to a low signal to noise ratio at ground station receiver. This is a typical consequence of very low transmitter RF power output, failures in downlink chain due to commercial off-the-shelf devices traditionally adopted in these missions, or mistakes in the process of assembly integration and verification of the satellite.
Existing HW or SW modems (mainly available from radio amateur community) are not designed and optimised to receive satellites signals; this means that low S/N ratios problem is worsened by Doppler and Doppler rate dynamics. Moreover, these modems are designed to operate in real-time on the incoming signals, so they cannot take benefits from the knowledge of the full data frame for optimised demodulation and decoding. Furthermore, because of the nature of amateur radio packet signal (i.e. data transmitted in short bursts), they do not coherently lock to the received signal (e.g. by using a PLL) but they demodulate it by using more lossy not-coherent techniques.
The present paper deals with the activities led in the framework of E-ST@R-II mission to decode the satellite TM signal, which was affected since launch by a power drop in the order of 20dB with respect to the computed link budget. Despite optimisation of the ground station, with installation of higher gain antennas and more performant LNA, it was not always possible to directly decode the received signal using existing modems, either HW or SW, and post-processing activities were necessary.
The E-ST@R-II signal is a standard radio-amateur AX.25 AFSK signal, consisting of a UHF carrier frequency, modulated by a subcarrier at 1700Hz, FSK modulated by the data stream (with +/-500Hz frequency deviation). In order to allow post-processing the signal, the RF spectrum around the carrier has been recorded by a Software-Defined-Radio (SDR) receiver providing I-Q samples files. At a first glance, the FM demodulated signal was obtained with existing free SWs, which allow some filtering and tuning of demodulator. Then, the subcarrier demodulation and data decoding have been performed by a dedicated algorithm. A coherent demodulation technique is applied and bit synchronisation is optimised over the duration of the full frame. The output are soft-decoded bits rather than a zeros-ones sequence as obtainable from other SWs. We also added the possibility to optimise the parameters of demodulator for better performance, e.g. based on eye-diagram tuning. The decoded soft-bits have been further processed knowing some expected pre-defined patterns in the received frame and knowing TM parameters ranges, with the objective to adjust the bits with the lower likelihood function to achieve a correct frame decoding or at least meaningful values for most important TM parameters. The described sequence of operations on the signals allows to partially decode packets from satellite in the first days after the launch, in order to assess its status and carry-out failure investigation concerning the drop of RF power. In the successive weeks, further analyses were carried out in order to improve the FM demodulator (e.g. to track Doppler and reduce S/N) and to optimise the bit-tuning algorithm. The self-developed post-processing decoded allowed an increment of the 150% of the decoded packets with respect to the direct decoded ones, deeply increasing the level of success of the E-ST@R-II mission because analysis on the housekeeping and payload data has been correctly conducted by the operators at the ground segment.
Existing HW or SW modems (mainly available from radio amateur community) are not designed and optimised to receive satellites signals; this means that low S/N ratios problem is worsened by Doppler and Doppler rate dynamics. Moreover, these modems are designed to operate in real-time on the incoming signals, so they cannot take benefits from the knowledge of the full data frame for optimised demodulation and decoding. Furthermore, because of the nature of amateur radio packet signal (i.e. data transmitted in short bursts), they do not coherently lock to the received signal (e.g. by using a PLL) but they demodulate it by using more lossy not-coherent techniques.
The present paper deals with the activities led in the framework of E-ST@R-II mission to decode the satellite TM signal, which was affected since launch by a power drop in the order of 20dB with respect to the computed link budget. Despite optimisation of the ground station, with installation of higher gain antennas and more performant LNA, it was not always possible to directly decode the received signal using existing modems, either HW or SW, and post-processing activities were necessary.
The E-ST@R-II signal is a standard radio-amateur AX.25 AFSK signal, consisting of a UHF carrier frequency, modulated by a subcarrier at 1700Hz, FSK modulated by the data stream (with +/-500Hz frequency deviation). In order to allow post-processing the signal, the RF spectrum around the carrier has been recorded by a Software-Defined-Radio (SDR) receiver providing I-Q samples files. At a first glance, the FM demodulated signal was obtained with existing free SWs, which allow some filtering and tuning of demodulator. Then, the subcarrier demodulation and data decoding have been performed by a dedicated algorithm. A coherent demodulation technique is applied and bit synchronisation is optimised over the duration of the full frame. The output are soft-decoded bits rather than a zeros-ones sequence as obtainable from other SWs. We also added the possibility to optimise the parameters of demodulator for better performance, e.g. based on eye-diagram tuning. The decoded soft-bits have been further processed knowing some expected pre-defined patterns in the received frame and knowing TM parameters ranges, with the objective to adjust the bits with the lower likelihood function to achieve a correct frame decoding or at least meaningful values for most important TM parameters. The described sequence of operations on the signals allows to partially decode packets from satellite in the first days after the launch, in order to assess its status and carry-out failure investigation concerning the drop of RF power. In the successive weeks, further analyses were carried out in order to improve the FM demodulator (e.g. to track Doppler and reduce S/N) and to optimise the bit-tuning algorithm. The self-developed post-processing decoded allowed an increment of the 150% of the decoded packets with respect to the direct decoded ones, deeply increasing the level of success of the E-ST@R-II mission because analysis on the housekeeping and payload data has been correctly conducted by the operators at the ground segment.
Attendee76
Cnes
Myriade Evolutions S-Band Transponder, the development story of a new equipment family
12:20 PM - 12:40 PMAbstract Submission
Heir of the Myriade family, Myriade Evolutions is the CNES new family of equipment for mini and micro satellites, developed in cooperation with Airbus Defense & Space (ADS) and Thales Alénia Space (TAS). Among the equipment developed for this project, the TM/TC Transceiver is the outcome of several years of CNES R&T actions and demonstrator development.
These series of R&T actions have begun when the first historical Myriade satellite was still under development to prepare the next generation of equipment. In the first time, two major axes of study where explored:
- The choice of components,
- The choice of modulation and coding considering the standardization evolution.
The equipment developed for the historical Myriade family were mainly based on the use of COTS components, with a strategy of qualification at equipment level instead of at component level. For example, there was 14 Qualification Models for the historical Myriade Transceiver. The TC link ground-to-board interface for Myriade was not a standard one, limiting therefore its capacity of inter-operability with other agency ground stations
The new choice of the ground-to-board interface was made by iterations, taking into account several modulations and coding schemes during the first steps of the R&T actions: GMSK, QPSK, OQPSK, with or without convolutional coding, with or without puncturing for the Telemetry link for example. The use of numerical circuit (FPGA) was also integrated. Indeed, for the historical Myriade Transceiver, the coding and modulation of the signal was managed by analogic or low level logical circuits. Thanks to these iterations of development, the difficulties presented by some coding schemes (internal clock management for puncturing codes for example) and some modulation (GMSK receiver – even if it is possible to use an OQPSK demodulator) have been identified.
Therefore, there was a convergence for a simple and standardized ground-to-board interface:
- For the TM Link: filtered QPSK, with convolutional coding (7,1/2), with or without differential pre-coding
- For the TC link: SP-L/PM modulation at 8 to 256 kbit/s with a classical frequency sweep process made by the Ground Station for the link establishment.
As the last R&T actions were beginning to develop a first prototype of equipment, CNES proposed a specific numerical architecture. This new architecture does not need any synchronization of the FPGA processing with the TM link data rate. Classically, this synchronization is made through an external PLL which is therefore not required. Indeed, the PLL circuit in generally sensitive to radiation environment and a component qualification was therefore avoided. This last R&T actions was made by Syrlinks and a joint patent was made concerning this specific numerical architecture. With this architecture, it is possible to manage every kind of signal as long as the symbol rate at the output of the modulator is a sub-multiple of the input data rate (it works for QPSK, OQPSK, QPSK with (7,1/2) coding, even OQPSK with (7,1/2) coding, 4D-TCM-8PSK at 2 bit/s/Hz, SP-L/PM and even PCM/PSK/PM).
After this last R&T action, a demonstrator was developed, including a transparent ranging function and a coherency mode, enabling to have a Transponder. The ranging function was developed in cooperation with ESA through a GSTP contract.
For the Myriade Evolutions invitation to tender, several proposals were received but the most interesting one was the Syrlinks proposal, based on the CNES R&T and demonstrator. Instead of COTS components (like with the historical Myriade equipment), ECSS class 3 components Quality was part of Syrlinks proposal.
The equipment is currently in the end of the delivery process and the two first missions that will use the Myriade Evolutions Transceiver/Transponder are MERLIN and MICROCARB.
MICROCARB is a CNES LEO mission; its main purpose is to map sources and sink of CO2 on a global scale. The launch of this satellite is scheduled in 2021. MICROCARB is part of the historical Myriade family, but includes some of Myriade Evolutions equipment (S and X-Band equipment).
MERLIN is a CNES/DLR LEO mission; its main purpose is to measure concentrations of atmospheric methane. The launch of this satellite is scheduled in 2024. It will be the first Myriade Evolutions mission.
In the first part of this article, the R&T and Demonstrator evolution, leading to the Myriade Evolutions Transceiver/Transponder, will be presented. Then, the new numerical architecture included in this equipment will be detailed. Finally, the main performances of the equipment, measured in CNES laboratory, will be presented.
These series of R&T actions have begun when the first historical Myriade satellite was still under development to prepare the next generation of equipment. In the first time, two major axes of study where explored:
- The choice of components,
- The choice of modulation and coding considering the standardization evolution.
The equipment developed for the historical Myriade family were mainly based on the use of COTS components, with a strategy of qualification at equipment level instead of at component level. For example, there was 14 Qualification Models for the historical Myriade Transceiver. The TC link ground-to-board interface for Myriade was not a standard one, limiting therefore its capacity of inter-operability with other agency ground stations
The new choice of the ground-to-board interface was made by iterations, taking into account several modulations and coding schemes during the first steps of the R&T actions: GMSK, QPSK, OQPSK, with or without convolutional coding, with or without puncturing for the Telemetry link for example. The use of numerical circuit (FPGA) was also integrated. Indeed, for the historical Myriade Transceiver, the coding and modulation of the signal was managed by analogic or low level logical circuits. Thanks to these iterations of development, the difficulties presented by some coding schemes (internal clock management for puncturing codes for example) and some modulation (GMSK receiver – even if it is possible to use an OQPSK demodulator) have been identified.
Therefore, there was a convergence for a simple and standardized ground-to-board interface:
- For the TM Link: filtered QPSK, with convolutional coding (7,1/2), with or without differential pre-coding
- For the TC link: SP-L/PM modulation at 8 to 256 kbit/s with a classical frequency sweep process made by the Ground Station for the link establishment.
As the last R&T actions were beginning to develop a first prototype of equipment, CNES proposed a specific numerical architecture. This new architecture does not need any synchronization of the FPGA processing with the TM link data rate. Classically, this synchronization is made through an external PLL which is therefore not required. Indeed, the PLL circuit in generally sensitive to radiation environment and a component qualification was therefore avoided. This last R&T actions was made by Syrlinks and a joint patent was made concerning this specific numerical architecture. With this architecture, it is possible to manage every kind of signal as long as the symbol rate at the output of the modulator is a sub-multiple of the input data rate (it works for QPSK, OQPSK, QPSK with (7,1/2) coding, even OQPSK with (7,1/2) coding, 4D-TCM-8PSK at 2 bit/s/Hz, SP-L/PM and even PCM/PSK/PM).
After this last R&T action, a demonstrator was developed, including a transparent ranging function and a coherency mode, enabling to have a Transponder. The ranging function was developed in cooperation with ESA through a GSTP contract.
For the Myriade Evolutions invitation to tender, several proposals were received but the most interesting one was the Syrlinks proposal, based on the CNES R&T and demonstrator. Instead of COTS components (like with the historical Myriade equipment), ECSS class 3 components Quality was part of Syrlinks proposal.
The equipment is currently in the end of the delivery process and the two first missions that will use the Myriade Evolutions Transceiver/Transponder are MERLIN and MICROCARB.
MICROCARB is a CNES LEO mission; its main purpose is to map sources and sink of CO2 on a global scale. The launch of this satellite is scheduled in 2021. MICROCARB is part of the historical Myriade family, but includes some of Myriade Evolutions equipment (S and X-Band equipment).
MERLIN is a CNES/DLR LEO mission; its main purpose is to measure concentrations of atmospheric methane. The launch of this satellite is scheduled in 2024. It will be the first Myriade Evolutions mission.
In the first part of this article, the R&T and Demonstrator evolution, leading to the Myriade Evolutions Transceiver/Transponder, will be presented. Then, the new numerical architecture included in this equipment will be detailed. Finally, the main performances of the equipment, measured in CNES laboratory, will be presented.
Attendee103
ESA
ESOC Ground Station Architecture for OPS-SAT
12:40 PM - 1:00 PMAbstract Submission
The SMILE Ground Station is located at the European Space Operations Centre (ESOC) in Darmstadt, Germany and is part of the Special Mission Infrastructure Laboratory Environment (SMILE). It currently comprises two terminals, ESOC-1, which is a commercial 3.7 meter S/X-Band antenna, and ESOC-1A, which supports the VHF, UHF and S-Band amateur radio frequency bands.
ESOC-1 is capable of transmitting and receiving in S-Band and receiving in X-Band. It is designed for tracking spacecraft in LEO and it features a 3 axis positioner which eliminates the "key hole" problem for high elevation passes. To protect the system from the environment, it is protected by a glass fibre radome. A fiber optic link connects the antenna to the back end and control room.
ESOC-1A features two crossed Yagi antennas, one for VHF and one for UHF, a 1.2 meter mesh dish for S-Band, all mounted on the same axis.
The back end consists of a CORTEX Command, Ranging and Telemetry Unit and a set of Software Defined Radios (SDR) for experimental modulation and communication protocols.
OPS-SAT is a ESA driven cubesat mission which is to launch in late 2019, it will utilize the UHF system of ESOC-1A with a dedicated GomSpace GS100 modem, as well as the S-Band system of ESOC-1 for TT&C. X-Band will be used for high bandwidth experimenter data. The MCS is based on SCOS-2000 and can operate interchangeably in UHF and S-Band.
The main mission of OPS-SAT is to allow external institutions to develop custom applications and take control of the spacecraft, including experiments with the communication systems. This flexibility is a perfect match for the SDR back end of ESOC-1.
ESOC-1 is capable of transmitting and receiving in S-Band and receiving in X-Band. It is designed for tracking spacecraft in LEO and it features a 3 axis positioner which eliminates the "key hole" problem for high elevation passes. To protect the system from the environment, it is protected by a glass fibre radome. A fiber optic link connects the antenna to the back end and control room.
ESOC-1A features two crossed Yagi antennas, one for VHF and one for UHF, a 1.2 meter mesh dish for S-Band, all mounted on the same axis.
The back end consists of a CORTEX Command, Ranging and Telemetry Unit and a set of Software Defined Radios (SDR) for experimental modulation and communication protocols.
OPS-SAT is a ESA driven cubesat mission which is to launch in late 2019, it will utilize the UHF system of ESOC-1A with a dedicated GomSpace GS100 modem, as well as the S-Band system of ESOC-1 for TT&C. X-Band will be used for high bandwidth experimenter data. The MCS is based on SCOS-2000 and can operate interchangeably in UHF and S-Band.
The main mission of OPS-SAT is to allow external institutions to develop custom applications and take control of the spacecraft, including experiments with the communication systems. This flexibility is a perfect match for the SDR back end of ESOC-1.
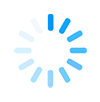