Session 8B1: Radioscience and Propagation
Tracks
H-IV
Thursday, September 26, 2019 |
2:00 PM - 3:40 PM |
Speaker
Attendee144
ESA
The implementation of a Radio-Science capability in the ESA tracking Network of Antennae
2:00 PM - 2:20 PMAbstract Submission
The European Space Agency operates its interplanetary spacecraft from an operations control centre located in Germany (ESOC). All probes are continuously tracked using a network of deep space ground 35-m antennae, located in Malargüe (Argentina), Cebreros (Spain) and New Norcia (Western Australia).
In recent years, one driver for the evolution of such network has been the possibility to support Radio-Science experiments, starting with the ones planned for the BepiColombo and JUICE missions. These experiments rely on an extremely accurate tracking system for both integrated Doppler and ranging observables, with a performance that is one order of magnitude better than what is currently available with X-band based tracking systems. The expected accuracy of this novel tracking system is 3 µm/s at 1000 s integration time and 20 cm for range-rate and range measurements, respectively.
In order to attain such improved level of spacecraft tracking accuracy, four major steps are to be undertaken at the ground station:
1) The use of a multi-frequency link with simultaneous transmissions of signal in X and Ka band and the reception of three signals, with the following scheme: X/X, X/Ka and Ka/Ka
2) The calibration of the slant tropospheric wet-delay, by means of a dedicated instrument based on a highly stable microwave radiometer
3) The use of a wideband ranging system allowing for a much improved jitter performance
4) The continuous calibration of the ground antenna delay
The first Deep Space Antenna (DSA) that will be equipped with such novel capability is DSA-3 in Malargüe (Argentina), positioned at an optimal geographical location in terms of propagation effects and simultaneous use for spacecraft operations and navigation. The provisions made during the design phase of DSA-3 for later Radio-Science upgrades are facilitating a smooth implementation of the new capability.
This paper describes the steps taken over the last decade to implement the Radio-Science capability, the underlying key requirements, the overall system design adopted in Malargüe, the main novel elements, the current status of the system implementation, and the roadmap to support the first Radio-Science experiments with BepiColombo in 2021 and the JUICE Jovian Orbit Insertion in 2029.
In recent years, one driver for the evolution of such network has been the possibility to support Radio-Science experiments, starting with the ones planned for the BepiColombo and JUICE missions. These experiments rely on an extremely accurate tracking system for both integrated Doppler and ranging observables, with a performance that is one order of magnitude better than what is currently available with X-band based tracking systems. The expected accuracy of this novel tracking system is 3 µm/s at 1000 s integration time and 20 cm for range-rate and range measurements, respectively.
In order to attain such improved level of spacecraft tracking accuracy, four major steps are to be undertaken at the ground station:
1) The use of a multi-frequency link with simultaneous transmissions of signal in X and Ka band and the reception of three signals, with the following scheme: X/X, X/Ka and Ka/Ka
2) The calibration of the slant tropospheric wet-delay, by means of a dedicated instrument based on a highly stable microwave radiometer
3) The use of a wideband ranging system allowing for a much improved jitter performance
4) The continuous calibration of the ground antenna delay
The first Deep Space Antenna (DSA) that will be equipped with such novel capability is DSA-3 in Malargüe (Argentina), positioned at an optimal geographical location in terms of propagation effects and simultaneous use for spacecraft operations and navigation. The provisions made during the design phase of DSA-3 for later Radio-Science upgrades are facilitating a smooth implementation of the new capability.
This paper describes the steps taken over the last decade to implement the Radio-Science capability, the underlying key requirements, the overall system design adopted in Malargüe, the main novel elements, the current status of the system implementation, and the roadmap to support the first Radio-Science experiments with BepiColombo in 2021 and the JUICE Jovian Orbit Insertion in 2029.
Attendee119
RPG Radiometer Physics GmbH
Accuracy and stability of atmospheric delay assessed by a RPG microwave radiometer with respect to ground calibration systems for ESA radioscience
2:20 PM - 2:40 PMAbstract Submission
Ground-based microwave radiometers can provide in line-of-sight real-time observations of tropospheric attenuation as well as wet and hydrostatic delay. While attenuation estimates enable the evaluation and control of communication link budgets, Flight Dynamics are interested in the tropospheric delay for calibrating Doppler measurements of spacecraft trajectories.
In the meteorological context, microwave radiometers measure the frequency dependent atmospheric emission and relate it to atmospheric variables like temperature and humidity profiles. The observed emission spectrum reflects the ability to absorb radiation. The fact that tropospheric attenuation of signals below 100 GHz is dominated by absorption, makes microwave radiometers a suitable tool for accurate attenuation measurements.
The standard RPG radiometer HATPRO offers 14 channels between 22 and 58 GHz. The channel frequencies are located along the water vapor line centered at about 22 GHz (K-band) and the oxygen absorption complex at around 60 GHz (V-band).
Each single channel directly provides an accurate attenuation measurement at the given channel frequency. Even more, the 14 HATPRO channels are sufficient to capture the complete information content of the attenuation spectrum between 1 and 100 GHz.
In contrast to tropospheric attenuation, the delay is not directly related to absorption. However, the Kramers-Kronig relation allows for retrieving it from microwave radiometer measurements as well. While the attenuation is strongly frequency-dependent, the tropospheric delay retrieved from HATPRO observations is valid for a wide range of frequencies (1-100 GHz, with negligible dispersion).
The highly variable wet delay is mainly driven by the column amount of water vapor (Integrated Water Vapor, IWV). The wet delay itself, does almost not depend on the column amount of cloud liquid (Liquid Water Path, LWP), but the observed absorption does. Therefore, single channel radiometers are not feasible for wet delay observations since they have no means to attribute measured emission signals to either LWP (with not significant delay effect) or IWV (the driver of wet delay).
On top, the absorption by atmospheric water does not completely depend on the number concentration of vapor and liquid molecules, but also on the emission temperature. This effect is captured by the RPG-HATPRO through its seven V-band channels. This additional information improves the accuracy of the wet component and enables the synchronous measurement of the hydrostatic component.
During the last decade, RPG could steadily improve the performance of attenuation and delay observations due to the ongoing development of receiver hardware and retrieval techniques. Nowadays, with radiometer Generation 5 (G5), both attenuation and delay are retrieved from Artificial Neural Network (ANN) algorithms, which use all 14 HATPRO channels as input. The ANNs are trained on the basis of several thousand vertical profiles of temperature, humidity, and cloud liquid from balloon soundings and a ECMWF model re-analysis.
The authors will assess the sensitivity and accuracy of retrieved attenuation and delay via so-called retrieval self-tests. Those self-tests quantify the retrieval’s ability to extract an atmospheric variable from a set of simulated brightness temperatures. Results demonstrate that the performance is related to both, the receiver noise characteristic and a scene-dependent retrieval error. The G5 performance is set into relation with the performance that was achieved with previous instrument generations.
When tropospheric delay observations are used for the calibration of Doppler measurements, the long-term stability is of major interest. The system stability is quantified by calculating the Allan Standard Deviation (ASD) in dependence of the Doppler observation time.
The instrumental contribution to the delay stability is extracted by a differential method: Two identical RPG-HATPRO-G5 radiometers have been deployed just a few meters from each other. ASD is calculated from the time series of the wet delay difference between them. The contribution of the retrieval algorithm due to a scene-dependent retrieval error is addressed by a worst-case analysis: The major impact on stability results from a sudden occurrence of clouds during the Doppler observation time.
Mounted on the optional azimuth turn-table, the RPG-HATPRO-G5 gain full sky scanning capabilities, which are, e.g. required for tracking spacecraft or astronomical objects. A data server tool being part of RPG-HATPRO’s operating software provides real-time delay measurements via a TCP/IP interface upon request. The pointing may be controlled by a tracking files that includes a list of elevation and azimuth pointing angles. This operational mode is applied within an ongoing ESA ESTEC development project, which uses an RPG-HATPRO-G5 as an integral part of a TDCS (Tropospheric Delay Calibration System) prototype.
In the meteorological context, microwave radiometers measure the frequency dependent atmospheric emission and relate it to atmospheric variables like temperature and humidity profiles. The observed emission spectrum reflects the ability to absorb radiation. The fact that tropospheric attenuation of signals below 100 GHz is dominated by absorption, makes microwave radiometers a suitable tool for accurate attenuation measurements.
The standard RPG radiometer HATPRO offers 14 channels between 22 and 58 GHz. The channel frequencies are located along the water vapor line centered at about 22 GHz (K-band) and the oxygen absorption complex at around 60 GHz (V-band).
Each single channel directly provides an accurate attenuation measurement at the given channel frequency. Even more, the 14 HATPRO channels are sufficient to capture the complete information content of the attenuation spectrum between 1 and 100 GHz.
In contrast to tropospheric attenuation, the delay is not directly related to absorption. However, the Kramers-Kronig relation allows for retrieving it from microwave radiometer measurements as well. While the attenuation is strongly frequency-dependent, the tropospheric delay retrieved from HATPRO observations is valid for a wide range of frequencies (1-100 GHz, with negligible dispersion).
The highly variable wet delay is mainly driven by the column amount of water vapor (Integrated Water Vapor, IWV). The wet delay itself, does almost not depend on the column amount of cloud liquid (Liquid Water Path, LWP), but the observed absorption does. Therefore, single channel radiometers are not feasible for wet delay observations since they have no means to attribute measured emission signals to either LWP (with not significant delay effect) or IWV (the driver of wet delay).
On top, the absorption by atmospheric water does not completely depend on the number concentration of vapor and liquid molecules, but also on the emission temperature. This effect is captured by the RPG-HATPRO through its seven V-band channels. This additional information improves the accuracy of the wet component and enables the synchronous measurement of the hydrostatic component.
During the last decade, RPG could steadily improve the performance of attenuation and delay observations due to the ongoing development of receiver hardware and retrieval techniques. Nowadays, with radiometer Generation 5 (G5), both attenuation and delay are retrieved from Artificial Neural Network (ANN) algorithms, which use all 14 HATPRO channels as input. The ANNs are trained on the basis of several thousand vertical profiles of temperature, humidity, and cloud liquid from balloon soundings and a ECMWF model re-analysis.
The authors will assess the sensitivity and accuracy of retrieved attenuation and delay via so-called retrieval self-tests. Those self-tests quantify the retrieval’s ability to extract an atmospheric variable from a set of simulated brightness temperatures. Results demonstrate that the performance is related to both, the receiver noise characteristic and a scene-dependent retrieval error. The G5 performance is set into relation with the performance that was achieved with previous instrument generations.
When tropospheric delay observations are used for the calibration of Doppler measurements, the long-term stability is of major interest. The system stability is quantified by calculating the Allan Standard Deviation (ASD) in dependence of the Doppler observation time.
The instrumental contribution to the delay stability is extracted by a differential method: Two identical RPG-HATPRO-G5 radiometers have been deployed just a few meters from each other. ASD is calculated from the time series of the wet delay difference between them. The contribution of the retrieval algorithm due to a scene-dependent retrieval error is addressed by a worst-case analysis: The major impact on stability results from a sudden occurrence of clouds during the Doppler observation time.
Mounted on the optional azimuth turn-table, the RPG-HATPRO-G5 gain full sky scanning capabilities, which are, e.g. required for tracking spacecraft or astronomical objects. A data server tool being part of RPG-HATPRO’s operating software provides real-time delay measurements via a TCP/IP interface upon request. The pointing may be controlled by a tracking files that includes a list of elevation and azimuth pointing angles. This operational mode is applied within an ongoing ESA ESTEC development project, which uses an RPG-HATPRO-G5 as an integral part of a TDCS (Tropospheric Delay Calibration System) prototype.
Attendee126
University Of Bologna
Tropospheric Delay Calibration System (TDCS): design and performances of a new generation of micro-wave radiometers for ESA deep space ground stations
2:40 PM - 3:00 PMAbstract Submission
Precise radiometric tracking is of key importance during operations of interplanetary missions and for radio science applications. Most of the recent deep space missions, including Cassini, Bepi-Colombo, and the upcoming JUICE mission, rely on a combination of X and Ka band radio link to mitigate the dispersive effects of propagation through interplanetary plasma, solar corona and Earth ionosphere, leaving tropospheric delay as one of the main error sources.
To meet the demanding requirements of the MORE and 3GM radioscience experiments onboard the Bepi-Colombo and JUICE missions, in terms of radiometric tracking accuracy and end-to-end frequency stability, ground-based microwave radiometers (MWR) are deemed as the most appropriate instruments for tropospheric delay calibration.
The system that is presented here represents the prototype of a new instrument for the calibration of tropospheric delay effects for ranging and radioscience at ESA Deep Space ground stations. This prototype is being developed in an ESA TRP contract as a JUICE Technological Development The methods, design, validation and setup of this prototype are the results of previous ESA studies, namely AWARDS and ASTRA. It is based also on the results obtained with the instruments for tropospheric correction developed by JPL in the late 1990s for CASSINI radioscience experiments.
The Tropospheric Delay Calibration System (TDCS), is a combination of instruments, software tools and procedures that allow the accurate estimation of the tropospheric delay along the direction of the link to the spacecraft and the derivation of tropospheric delay rate by minimizing the effect of instability of the instrument.
The products of this system are used to correct the ranging and integrated Doppler observables for radioscience experiment and spacecraft navigation.
The primary component of TDCS is an ultra-stable MWR unit, which is based on the RPG HATPRO-G5 instrument and has been upgraded to incorporate new functionalities (the details of this instrument are described in another paper submitted to this workshop).
These upgrades include, among others, the addition of an Azimuth turn-table to gain full sky scanning capabilities for spacecraft tracking and the integration of a 1.2 m parabolic reflector to narrow the beam with of the MWR and reduce the solar intrusion during periods of superior solar conjunction.
Operations and control of the radiometer are performed through an External Control System (ECS), which is a modified version of an RPG standard software tool and communicates with the MWR using a dedicated optical-fibre line.
Finally, the interface between the ground station Front-End Controller (FEC) and the MWR ECS is managed using the Calibration, data Acquisition and Processing System (CAPS) software tool developed by the University of Bologna, which is responsible for high-level monitoring and controlling functions and for the generation of CSP calibration cards using the delay data collected by the MWR to be submitted to the processing system of ESA Flight Dynamic.
Installation of the first TDCS prototype was performed in February 2019 at the DS3 station complex in Malargue. Results of the installation campaign are presented here, with details on the platform selection process, installation procedures and test results.
This paper presents TDCS performance results, in particular the ones obtained during the test campaign performed with the GAIA spacecraft after deployment at DS3. The advantages of using GAIA as test bed are the good knowledge of its dynamical model and its orbit around the second Lagrange point, which avoids any Doppler and ranging data degradation due to solar plasma. Radiometric observables collected by the ground station are processed in an orbit determination (OD) filter, which includes detailed physical models of the GAIA spacecraft, its operating environment and ancillary information on spacecraft attitude and maneuvers.
The purpose of this end-to-end test is not to reproduce the full OD process of the ESA flight dynamics team, but to validate TDCS products by comparing the observable residuals with those obtained using standard tropospheric calibrations from GNSS receivers and current atmospheric profilers.
To meet the demanding requirements of the MORE and 3GM radioscience experiments onboard the Bepi-Colombo and JUICE missions, in terms of radiometric tracking accuracy and end-to-end frequency stability, ground-based microwave radiometers (MWR) are deemed as the most appropriate instruments for tropospheric delay calibration.
The system that is presented here represents the prototype of a new instrument for the calibration of tropospheric delay effects for ranging and radioscience at ESA Deep Space ground stations. This prototype is being developed in an ESA TRP contract as a JUICE Technological Development The methods, design, validation and setup of this prototype are the results of previous ESA studies, namely AWARDS and ASTRA. It is based also on the results obtained with the instruments for tropospheric correction developed by JPL in the late 1990s for CASSINI radioscience experiments.
The Tropospheric Delay Calibration System (TDCS), is a combination of instruments, software tools and procedures that allow the accurate estimation of the tropospheric delay along the direction of the link to the spacecraft and the derivation of tropospheric delay rate by minimizing the effect of instability of the instrument.
The products of this system are used to correct the ranging and integrated Doppler observables for radioscience experiment and spacecraft navigation.
The primary component of TDCS is an ultra-stable MWR unit, which is based on the RPG HATPRO-G5 instrument and has been upgraded to incorporate new functionalities (the details of this instrument are described in another paper submitted to this workshop).
These upgrades include, among others, the addition of an Azimuth turn-table to gain full sky scanning capabilities for spacecraft tracking and the integration of a 1.2 m parabolic reflector to narrow the beam with of the MWR and reduce the solar intrusion during periods of superior solar conjunction.
Operations and control of the radiometer are performed through an External Control System (ECS), which is a modified version of an RPG standard software tool and communicates with the MWR using a dedicated optical-fibre line.
Finally, the interface between the ground station Front-End Controller (FEC) and the MWR ECS is managed using the Calibration, data Acquisition and Processing System (CAPS) software tool developed by the University of Bologna, which is responsible for high-level monitoring and controlling functions and for the generation of CSP calibration cards using the delay data collected by the MWR to be submitted to the processing system of ESA Flight Dynamic.
Installation of the first TDCS prototype was performed in February 2019 at the DS3 station complex in Malargue. Results of the installation campaign are presented here, with details on the platform selection process, installation procedures and test results.
This paper presents TDCS performance results, in particular the ones obtained during the test campaign performed with the GAIA spacecraft after deployment at DS3. The advantages of using GAIA as test bed are the good knowledge of its dynamical model and its orbit around the second Lagrange point, which avoids any Doppler and ranging data degradation due to solar plasma. Radiometric observables collected by the ground station are processed in an orbit determination (OD) filter, which includes detailed physical models of the GAIA spacecraft, its operating environment and ancillary information on spacecraft attitude and maneuvers.
The purpose of this end-to-end test is not to reproduce the full OD process of the ESA flight dynamics team, but to validate TDCS products by comparing the observable residuals with those obtained using standard tropospheric calibrations from GNSS receivers and current atmospheric profilers.
Attendee104
LSE Space GmbH for ESA - European Space Agency
Evaluating the radio frequency interference potential of 5G mobile networks on space links at millimeter waves
3:00 PM - 3:20 PMAbstract Submission
In the framework of the World Radiocommunication Conference 2019 (WRC-19) of the International Telecommunication Union (ITU), Agenda Item 1.13 (AI 1.13) will address the identification and the possible additional allocations of radio-frequency spectrum to the mobile services on a primary basis. Such identifications and allocations are required to serve the future development of the International Mobile Telecommunications (IMT) for 2020 and beyond, mainly focused on systems supporting the fifth generation (5G) of cellular mobile communications. The frequency range of interest goes from 24.25 to 86 GHz, which fully covers all the high-frequency bands used or planned by the European Space Agency’s space missions for high data rate transmissions. The use of frequencies at millimeter waves is a key interest of the agency, as these high frequency bands are not as congested as the lower bands and they provide several performance advantages. Hence, the need of ensuring a proper protection from the future IMT systems in terms of received radio frequency interference (RFI) has become one of the crucial tasks of the ESA’s frequency management office in preparation for the upcoming WRC-19.
In addition to data transmission from space, it is necessary to ensure the protection of the adjacent frequency bands used by many earth observation missions equipped with passive sensors. The impact of high levels of unwanted emissions from IMT-2020 devices is a potential threat for such sensitive instruments and could compromise the data quality of future missions.
This contribution shows the potential impact of IMT deployments presenting an in-band spectral overlap with ESA’s missions using or planning to use frequency bands allocated to the Earth Exploration-Satellite Service (EESS) and to the Space Research Service (SRS) as follow:
• 25.5-27.0 GHz, allocated to EESS (space-to-Earth) and SRS (space-to-Earth) – already used by EDRS and planned for use by EUCLID, PLATO, WFIRST, MTG, MetOp-SG and many other missions in the near future;
• 31.8-32.3 GHz, allocated to SRS Deep Space (space-to-Earth) – already used by BepiColombo, Hayabusa2 and several NASA missions, and planned for Juice;
• 37.0-38.0 GHz, allocated to SRS (space-to-Earth) – earmarked for future lunar manned missions like the international moon village.
To ensure RFI free operations of the receiving earth stations operated by ESA and other space agencies, it is necessary that the emissions of IMT-2020 mobile networks be compliant with the relevant ITU recommended protection criteria for SRS and EESS stations. The criteria mainly depend on the mission type and on the sensitivity of the receiver and are usually expressed as maximum permitted interference density level received by the victim for a given probability. Particular focus is given to the ESA’s tracking network and to the ground station sites located in New Norcia (Australia), Cebreros (Spain), Malargüe Sur (Argentina) and Kiruna (Sweden). The high performance of the deep space stations makes them very sensitive to a potential interference source on ground. Results are shown in terms of required separation distances, i.e. the minimum distance between the earth station and the IMT station ensuring that the protection criteria for the earth station are met by the emissions of an IMT base station or user equipment.
Relevant expert groups provided the IMT network specifications, which were then formalized by the ITU study groups and universally adopted in compatibility studies. The IMT base stations will exploit Massive Multiple-Input & Multiple-Output (MIMO) techniques, able to serve more than one user at the same time by adopting beamforming antenna arrays with highly directional antennas. The IMT network topology and system characteristics differ among deployment scenarios and all possible combinations of them are considered in the RFI assessment.
It is to be noted that transmission media of radiowaves include all possible routes between a radio transmitter and a receiver consisting of free space and atmospheric propagation as well as electromagnetic interaction with the ground surface and surrounding medium. Received interference can derive from several propagation mechanisms including line-of-sight propagation, diffraction, scatter, ducting, reflection/refraction, etc. which strongly depend on climate, radio frequency, link availability, distance and path topography; at any time a single mechanism or more than one may be present. For this, the ESA analysis adopts the terrestrial propagation modelling of the latest version of Recommendation ITU-R P.452 “Prediction procedure for the evaluation of interference between stations on the surface of the Earth at frequencies above about 0.1 GHz” as the method to assess the interference potential between the IMT terrestrial networks and Earth-to-space receiving stations, and to ensure a satisfactory coexistence. Both static and dynamic scenarios are considered, together with several sensitivity analyses. Results point out the need of having separation distances up to 100 km depending on the station and frequency under consideration.
In addition to data transmission from space, it is necessary to ensure the protection of the adjacent frequency bands used by many earth observation missions equipped with passive sensors. The impact of high levels of unwanted emissions from IMT-2020 devices is a potential threat for such sensitive instruments and could compromise the data quality of future missions.
This contribution shows the potential impact of IMT deployments presenting an in-band spectral overlap with ESA’s missions using or planning to use frequency bands allocated to the Earth Exploration-Satellite Service (EESS) and to the Space Research Service (SRS) as follow:
• 25.5-27.0 GHz, allocated to EESS (space-to-Earth) and SRS (space-to-Earth) – already used by EDRS and planned for use by EUCLID, PLATO, WFIRST, MTG, MetOp-SG and many other missions in the near future;
• 31.8-32.3 GHz, allocated to SRS Deep Space (space-to-Earth) – already used by BepiColombo, Hayabusa2 and several NASA missions, and planned for Juice;
• 37.0-38.0 GHz, allocated to SRS (space-to-Earth) – earmarked for future lunar manned missions like the international moon village.
To ensure RFI free operations of the receiving earth stations operated by ESA and other space agencies, it is necessary that the emissions of IMT-2020 mobile networks be compliant with the relevant ITU recommended protection criteria for SRS and EESS stations. The criteria mainly depend on the mission type and on the sensitivity of the receiver and are usually expressed as maximum permitted interference density level received by the victim for a given probability. Particular focus is given to the ESA’s tracking network and to the ground station sites located in New Norcia (Australia), Cebreros (Spain), Malargüe Sur (Argentina) and Kiruna (Sweden). The high performance of the deep space stations makes them very sensitive to a potential interference source on ground. Results are shown in terms of required separation distances, i.e. the minimum distance between the earth station and the IMT station ensuring that the protection criteria for the earth station are met by the emissions of an IMT base station or user equipment.
Relevant expert groups provided the IMT network specifications, which were then formalized by the ITU study groups and universally adopted in compatibility studies. The IMT base stations will exploit Massive Multiple-Input & Multiple-Output (MIMO) techniques, able to serve more than one user at the same time by adopting beamforming antenna arrays with highly directional antennas. The IMT network topology and system characteristics differ among deployment scenarios and all possible combinations of them are considered in the RFI assessment.
It is to be noted that transmission media of radiowaves include all possible routes between a radio transmitter and a receiver consisting of free space and atmospheric propagation as well as electromagnetic interaction with the ground surface and surrounding medium. Received interference can derive from several propagation mechanisms including line-of-sight propagation, diffraction, scatter, ducting, reflection/refraction, etc. which strongly depend on climate, radio frequency, link availability, distance and path topography; at any time a single mechanism or more than one may be present. For this, the ESA analysis adopts the terrestrial propagation modelling of the latest version of Recommendation ITU-R P.452 “Prediction procedure for the evaluation of interference between stations on the surface of the Earth at frequencies above about 0.1 GHz” as the method to assess the interference potential between the IMT terrestrial networks and Earth-to-space receiving stations, and to ensure a satisfactory coexistence. Both static and dynamic scenarios are considered, together with several sensitivity analyses. Results point out the need of having separation distances up to 100 km depending on the station and frequency under consideration.
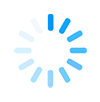