Tutorials
Tutorials will be held on Monday 20th April 2020
They will be located in the ESTEC Conference area in Newton rooms.
Register to attend the tutorials and the EFTF Conference
Tutorial fees (excluding local VAT):
|
|
Standard delegate |
€ 250 |
Student/retiree |
€ 100 |
Tutorial schedule:
Monday 20th April |
Tutorials - Track 1 |
Tutorials - Track 2 |
08:45 - 10:45 |
Pascale Defraigne (ORB) |
Eric Burt (JPL) |
10:45 - 11:00 |
Coffee / tea break |
Coffee / tea break |
11:00 - 13:00 |
Ashwin Seshia (Univ Cambridge) |
Nathan Newbury (NIST) |
13:00 - 14:00 |
Lunch break |
Lunch break |
14:00 - 16:00 |
Archita Hati (NIST) |
Anne Curtis |
16:00 – 16:15 |
Coffee / tea break |
Coffee / tea break |
16:15 - 18:15 |
Cesar Boatella Polo (ESA) + Gregory Weaver (JHU/APL) 2/Frequency Behaviour of Quartz Resonators Under Low Dose Exposures to Ionizing Radiation' |
Yann Le Coq (SYRTE) |
Tutorial Speakers:
Dr. Pascale Defraigne
GNSS and Two-Way time transfer
In this tutorial, we will describe the crucial role of timing in the Global Navigation Satellite Systems (GNSS, i.e. GPS, Galileo, Beidou and Galileo). We will then examine how from the GNSS measurements it is possible to synchronize clocks on the ground, and compare time and frequencies of remote clocks. In a second part the Two Way Satellite Time and Frequency Transfer technique will be also developed. The operation and performances of both techniques will be studied, their current limitations and possible improvements will be discussed.
Pascale Defraigne is Project Leader at the Royal Observatory of Belgium. She completed her PhD in Physics at the Université Catholique de Louvain (UCL), Belgium, in 1995, for studies on mantle convection and deformations of the Earth internal boundaries. She then moved to the Royal Observatory of Belgium to start research activities on GNSS time and frequency transfer, time scale generation, and ionospheric sounding based on GNSS measurements. Pascale Defraigne is deeply involved in the development and upgrade of the Galileo System for all what concerns the Galileo System Time. Among her involvement in international bodies, Pascale Defraigne assured the Presidency of the Commission 31 "TIME" of the International Astronomical Union (2006-2009) and since 2012 she assures the Chair of the CCTF Working Group on GNSS Time Transfer (CCTF being the Consultative Committee for Time and Frequency, sub-committee of the International Committee for Weights and Measurements). She was also member of the GNSS Science Advisory Committee of the European Space Agency (ESA) during 2012-2019.
Dr. Ashwin Seshia
MEMS oscillators
MEMS oscillators are increasingly being adopted for applications in timing and frequency control due to the consequent benefits of size, power dissipation, and ease of co-integration with CMOS technologies. In recent years, MEMS oscillator products are also starting to have an impact on applications demanding high accuracies. Measured quality factors for single-crystal silicon resonators now approach 10 million at room temperature while the power dissipation for precision oven-controlled MEMS oscillators is significantly lower than that for counterpart OCXOs based on traditional crystal technologies. This tutorial will commence with a background to historical developments in the field followed by an introduction to the design principles for MEMS oscillators. Topics relating to MEMS resonator design and fabrication, transduction principles, lumped element modelling, oscillator design, temperature sensitivity and temperature compensation, and packaging will be discussed with a focus on capacitive-gap transduced silicon MEMS oscillators that currently dominate the MEMS-based timing industry.
Ashwin Seshia received the B.Tech. degree in Engineering Physics from IIT Bombay in 1996, the M.S. and Ph.D. degrees in Electrical Engineering and Computer Sciences from the University of California at Berkeley, Berkeley, in 1999 and 2002, respectively, and the M.A. degree from the University of Cambridge in 2008. He joined the Faculty of the Engineering Department at the University of Cambridge in 2002, where he is currently the Professor of Microsystems Technology and a Fellow of Queens’ College. He heads the MEMS research group at the Cambridge University Nanoscience Centre, and is also a co-investigator of the Cambridge Centre for Smart Infrastructure and Construction. He has published over 200 peer-reviewed research papers in the area of MEMS and is a co-inventor on over 20 granted patents / patent applications, particularly in areas relating to linear and non-linear resonant microsystems, and applications to sensors and sensor systems. Ashwin is a Fellow of the Institute of Physics (IoP) and the Institution of Engineering and Technology (IET). He serves as Editor for the IEEE Journal of Microelectromechanical systems (JMEMS) and Associate Editor for the IEEE Transactions on Ultrasonics, Ferroelectrics and Frequency Control. He has previously served on the editorial boards for the IoP Journal of Micromechanics and Microengineering and the IEEE Transactions on Nanotechnology. Ashwin has also served on several conference program committees including the IEEE Frequency Control Symposium and the European Frequency and Time Forum, as well as the IEEE MEMS Conference and IEEE International Electron Devices Meeting. In 2018, he received the IEEE Sensors Technical Achievement Award (Advanced Career - Sensor Systems) "for pioneering contributions to resonant microsystems with application to sub-surface density contrast imaging and energy harvesting systems".
Dr. Archita Hati
Phase Noise Measurement Techniques
Noise, present everywhere, causes a signal source to deviate from its ideal performance. This noise introduces time dependent phase and amplitude fluctuations on the signal. The spectral purity of a frequency source can be characterized in terms of phase modulation (PM) and amplitude modulation (AM) noise. This tutorial will cover the basic theory of modulation noise, the origin of different noise types, and the effects of signal manipulation such as amplification, frequency translation, and multiplication on the spectral purity of a signal. Various phase noise measurement techniques will be discussed, in particular, advantages and drawbacks of the cross-spectrum technique.
Archita Hati received the M.Sc. degree in physics, the M.Phil. degree in microwaves, and the Ph.D. degree in physics from the University of Burdwan, India, in 1992, 1993, and 2001, respectively. She is currently an Electronics Engineer with the Time and Frequency Division, National Institute of Standards and Technology, Boulder, CO, USA, where she is the Calibration Service Leader of the Time and Frequency Metrology Group. Her current research interests include phase noise metrology, ultra-low noise frequency synthesis, development of low-noise microwave and opto-electronic oscillators, and vibration analysis. Dr. Hati was the recipient of the Allen V. Astin Measurement Science Award in 2015 for developing a world-leading program of research and measurement services in phase noise.
Dr. Gregory Weaver
Frequency Behavior of Quartz Resonators Under Low Dose Exposures to Ionizing Radiation
The radiation sensitivity of quartz has been studied for decades due to the crucial time and frequency control operations of many space flight systems. The instance of solar flares as well as orbital passage through regions like the South Atlantic Anomaly lead to concern for standalone quartz based frequency reference systems in satellites. This tutorial will conduct a review of the work of many contributors, such as M. Block, P. Cash, E. Seydel, J. C. King, J. J. Boy, and G. Cibiel. Their conclusions, as well as, experimental results from years of radiation experiments on precision SC-cut resonators conducted by the Johns Hopkins University Applied Physics Laboratory will be presented to inform the attendees on the curious idiosyncratic behavior of quartz resonators to ionizing radiation in the space environment.
Gregory L. Weaver is a member of the Principal Professional Staff of the Johns Hopkins University Applied Physics Laboratory (JHU/APL). He is the Program Manager for Positioning, Navigation, and Timing (PNT) within the JHU/APL Space Exploration Sector. He is a technologist and subject matter expert in both the technical and business aspects of timing and frequency control and has held positions as a senior research engineer, technical manager and marketing strategist. Mr. Weaver was the lead engineer for all JHU/APL Ultra-stable Oscillator projects since 2003, including most recently the Gravity Recovery and Climate Experiment Follow-on (GRACE-FO) mission. He has contributed frequently to a variety of technical proceedings including the IEEE International Frequency Control Symposium (IFCS), Precise Time and Time Interval Systems and Application Meeting (PTTI) and the European Frequency and Time Forum (EFTF). Mr. Weaver serves currently as the Editorial Chair of the IFCS.
Dr. Eric Burt
Microwave Atomic Clocks
Microwave atomic clocks are everywhere in society. They range from chip-scale models that can fit into hand-held electronics, to “hardened” units that are flown in space, to laboratory units that reach state of the art levels of precision and accuracy. The term “microwave” corresponds to the wavelength of the radiation used to stimulate the internal atomic transition that forms the basis of the clock. Atomic clock technology grew out of experiments in the 1930’s to investigate and characterize internal atomic structure. It was quickly realized that energy levels of certain internal atomic states, and so the frequency of electromagnetic radiation used to make transitions between them, are exquisitely stable and immune to external perturbations and so might be used as a reference for a frequency standard. The first atomic clocks were based on techniques used in these scientific investigations. Due to the intervening 80+ years of development, microwave atomic clocks enjoy a high degree of sophistication and technical maturity that enables their wide range of applications. In the first part of this tutorial I will give an overview of how atomic clocks work in general and then describe in detail the underlying physics. Crucial to building atomic clocks is being able to measure their performance, so I will also describe the various metrics used. In the second part of the tutorial, I will give several key examples that represent the range of microwave atomic clock applications. These will include highly engineered space clocks, ultra-stable trapped ion clocks, and accurate laser-cooled fountain clocks. By the end of the tutorial, attendees should be able to understand the strengths and weaknesses of the various clock technologies and to understand talks given at the conference on this topic.
Eric Burt received a B.S. degree with honors in mathematics from the University of Michigan, Ann Arbor, Michigan in 1979, a M.S. degree in physics from the University of Washington, Seattle, Washington in 1990 and a Ph.D. in physics from the University of Washington in 1995. His Ph.D. thesis, supervised by Prof. Warren Nagourney, was in the field of experimental atomic physics on the trapping and laser-cooling of single indium ions. From 1995 to 1997 he was a postdoctoral fellow at the University of Colorado, in Boulder, Colorado working with Carl Wieman and Eric Cornell on experiments with Bose-Einstein condensates including the first experiment to demonstrate a dual-species condensate and the first experiment to demonstrate higher-order (laser-like) coherence in condensate atoms. From 1997 to 2001 he worked at the U.S. Naval Observatory in Washington, D.C. developing a laser-cooled cesium fountain atomic clock. From 2001 to the present he has worked at the Jet Propulsion Laboratory, California Institute of Technology most recently as a Principal Member of Technical Staff. His work at JPL has included development of both ion and laser-cooled neutral atomic clocks and using atomic clocks to place limits on fundamental constant variation. Dr. Burt is a member of the American Physical Society, and a senior member of the IEEE. He is on the technical program committee for the IEEE Frequency Control Symposium and has served as both the chair of that committee and the general chair for the conference as well as vice-chair for group 3 (microwave atomic clocks). He has also served on the steering committee for the APS Topical Group on Precision Measurement and Fundamental Constants. He is currently the secretary treasurer for this topical group.
Dr. Nathan Newbury
Optical time-frequency transfer over fiber and free-space
Optically based methods of time-frequency transfer have been developed to support long distance connections between clocks over both fiber-optic networks and free-space (i.e. the air). As with optical clocks, these methods often exploit the high coherence possible with cavity-stabilized lasers and frequency combs. I will discuss and compare the approaches of optical time-frequency transfer over fiber optics and free space. The tutorial will discuss the basics of fiber-optic based methods, which are now well-developed and varied, and will then focus in more detail on recent work in free-space time-frequency transfer.
Nathan Newbury leads the Fiber Sources and Applications group in the Applied Physics Division at NIST. He also currently serves as the acting Division Chief. He received a Ph.D. from Princeton University in 1992 and has been at NIST since 2000. His research at NIST has focused on the development and application of fiber-laser-based frequency combs, with a recent emphasis on time-frequency transfer and dual-comb spectroscopy. He is a fellow of NIST and of the Optical Society (OSA).
Dr. Anne Curtis
Optical Atomic Clocks and Applications
Optical frequency standards based on atomic references are now demonstrating accuracies at the 10-18 level. With this level of accuracy and sensitivity, these clock systems can also be used as exquisite quantum sensors, with the ability keep track of more than just time and frequency. In this tutorial we will focus on laser-cooled ion- and neutral-atom-based frequency standards, and what it takes to evaluate and reduce systematic uncertainties in optical clocks to the 10-18 level and below. We will also discuss the methods by which direct frequency comparisons are being made between optical frequency standards around the globe, and how the data from these measurement campaigns are being used not only in the evaluation of clock systems, but also in tests of fundamental physics. We will finish by discussing applications of optical clock technology for geodesy and environmental monitoring, tests of the Standard Model and General Relativity, and how future space-based optical clocks will extend the frontier for frequency metrology, satellite navigation, and improved tests of fundamental physics.
Anne Curtis received her PhD from the Department of Physics at the University of Colorado, Boulder, USA. Her thesis research was conducted at the National Institute for Standards and Technology (NIST), Boulder, and focused on the neutral calcium optical frequency standard located there. She was then awarded a three-year Royal Society USA Research Fellowship enabling her to do postdoctoral research in the UK, where she worked with permanent-magnet-based atom chips for creating low-dimensional Bose-Einstein condensates at Imperial College London. At the end of the fellowship she joined the UK National Physical Laboratory (NPL) to initiate a project on a next-generation neutral atom frequency standard, this one made from strontium atoms held in a lattice trap. Her current research focuses on ion-based optical frequency standards, as well as molecular spectroscopy methods for detection of trace gases with relevance to industrial, environmental, and medical sectors.
Dr. Yann Le Coq
Optical frequency Combs for Frequency Metrology
In this tutorial, I will cover the basics of optical frequency combs, in the context of frequency metrology. I’ll introduce the physics, concepts and technical implementations related to femtosecond lasers for metrology, including the various characterization of noise processes relevant to optical frequency combs. I will explain how these lasers are used routinely to measure and compare optical frequency standards in research laboratories. I will give an overview of basic techniques necessary to optical frequency combs operation as high precision measuring devices such as phase lock loops, transfer oscillator techniques, counters, phase noise analysis,… I will also give some brief overview of more advanced topics related to on-going research and technologies in the domain.
Yann Le Coq graduated from Institut d’Optique as a research engineer in Optics in 1998, received his PhD in Physics from Université Paris Sud (Orsay) in 2002 and his Habilitation diploma in 2014 from Sorbonne Université. He joined NIST Boulder Optical Frequency Group in 2005-2006 where he worked on optical clocks and optical frequency combs, and was hired at LNE-SYRTE in 2007 where he has worked, since, on the subjects of optical frequency combs (including applications to optical clock and low phase noise sources) and ultra-stable lasers. Since 2018, in parallel to his research activity, he has been the director of the FIRST-TF Research Federation, which regroups the various structures working on Time and Frequency in France. He received the EFTF Young Scientist award in 2014 for his work on optical frequency combs.
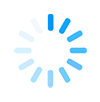